Assessing the Impact of Road and Land Use on Species Diversity of Trees, Shrubs, Herbs and Grasses in the Mountain Landscape in Southern Africa
- 1Department of Forest Engineering, Faculty of Agronomy and Forest Engineering, Eduardo Mondlane University, Maputo, Mozambique
- 2Department of Biological and Environmental Science, University of Jyvaskyla, Jyvaskyla, Finland
- 3N'Lab, Maputo, Mozambique
- 4Faculty of Agronomic and Biological Sciences, University of Púnguè, Manica, Mozambique
- 5Department of Biological Science, Faculty of Science, Eduardo Mondlane University, Maputo, Mozambique
Mountain landscape, described as a global biodiversity hotspot due to high endemism, is threatened by land-use change, including management and modification of vegetation. However, there is little knowledge about how road and land use affect plant diversity in mountains landscapes, particularly in southern Africa. Previous studies have studied the impact of the road or land use on plant species diversity separately and have concentrated on a single plant species. Here we compare the plant diversity of regenerated trees, shrubs, herbaceous plant, and grasses among Forest, Fallow, Agriculture, and Road in the Moribane Forest Reserve (MFR), in Eastern Chimanimani Mountain landscape in Mozambique. To assess how land-use change affects plant diversity, we conducted 45 transects along the roadside and randomly established 24 quadrats in the Agriculture fields and Fallow and 26 quadrats in the pristine Forest. In each transect and quadrats, we recorded the occurrence of four plant life forms (regenerated trees, shrubs, herbaceous, and grass species) to determine the alpha and beta-diversity across land-uses, and we assessed the invasiveness of each species. Species composition varied significantly among the land-uses types. Roadside had higher species diversity and the highest number of invasive species (138 total species of all plant life forms; 31 invasive species), following Agriculture (72; 30), Fallow (81; 20), and Forest (78; 19). There was no similarity in species between roadsides and other land-uses. Furthermore, roadside recorded the highest average species turnover for all plant life forms following Agriculture, Forest, and Fallow. Among the plants, the most important life form was herbaceous with 143 species, following grass with 86 species, shrubs with 86, and regenerated trees with 65 species. The land-use pattern makes the landscape more diversified in the study area and, as a result, increase the plant species richness and diversity by species replacement. This study is unique in collecting and analyzing data on different plant life forms on roadsides linked with a range of different land-use types within a small region of a mountain landscape in southern Africa.
Introduction
Southern Africa is known as one of the highly biodiverse regions in the world (Biggs et al., 2008), with most biodiversity hotspots concentrated in the mountainous landscapes (Spehn and Korner, 2010). The biodiversity, however, continues to decrease at unprecedented rates as human development and expansion lead to habitat loss and landscape fragmentation, which negatively affect biodiversity (Spehn et al., 2006; Slingenberg et al., 2009). The primary mechanism for protecting and maintaining biodiversity is conservation areas. However, in Mozambique, Conservation Areas cover only 26% of its territory. Nevertheless, despite the effort so far, the biodiversity loss continues to deepen as 96% of Conservation Areas have human settlements within their borders and surroundings (Givá, 2016). Still, extensive human activity within their boundaries can undermine this objective (Jones et al., 2018) induced by population growth.
One of the most cited causes of land-use change in southern Africa is shifting cultivation (also known as slash-and-burn agriculture). Shifting cultivation is a system of agriculture that involves cultivating a piece of land for a few years. Subsequently, leaving it uncultivated or fallow for a much more extended period so that the natural vegetation that develops on it restores the soil fertility that declined during cropping. The agricultural landscape in shifting cultivation areas consists of scattered cultivated fields and a mosaic of regrowth (fallow) vegetation in different stages of regeneration (Aweto, 2013). In most tropical regions, the fallow period lasts 20 to 30 years, allowing complete secondary forest regeneration (Poorter et al., 2016). However, the transition from shifting cultivation to more intensive land uses can negatively impact the environment, leading to permanent deforestation, biodiversity loss, and decline in forest productivity (van Vliet et al., 2012; Gogoi et al., 2020). Studies of succession after shifting cultivation in tropical regions have also indicated that the diversity of woody species gradually increases with fallow age. It is also likely that the frequent use of fire to prepare the fields for the following year's crops favors grasses, which probably limit seedling establishment due to competition (Gogoi et al., 2020).
Recent evidence suggests that transportation is particularly the primary human activity affecting, direct or indirectly, biodiversity in protected areas (Votsi et al., 2012; Fyumagwa et al., 2013; Helldin, 2019). Building and paving a road through a conservation area can increase access to areas previously not accessible and, therefore, may increase habitat loss, fragmentation, land-use change, and land-use intensification, since roads act as corridors for the entry of materials (Laurance and Balmford, 2013; Barber et al., 2014; Laurance et al., 2017). As human populations continue to increase, road density and land-use dynamic will likely increase as well (Bennett, 2017). Despite their impact on biodiversity, road plays a paramount role in developing and maintaining the economic activity that is vital for the quality of modern life and contributing to the overall fulfilment and social functioning of the community (Lugo and Gucinski, 2000; Alamgir et al., 2017). In southern Africa, road construction is one of the crucial factors for development, poverty alleviation and provision of socioeconomic opportunities, economic growth, improvement of quality of life, social wellbeing, and reach of the agricultural market (Dobson et al., 2010; Fyumagwa et al., 2013; Hopcraft et al., 2015).
A considerable amount of review literature has examined the impact of roads on biodiversity (Suárez-Esteban et al., 2016; Root-Bernstein and Svenning, 2018; Lázaro-Lobo and Ervin, 2019). These studies found that anthropogenic linear gaps such as roadsides can increase plant abundance and diversity, and act as corridors between habitat fragments, providing continuous links of habitat that channel the movement of fauna and flora between geographically distant areas. In particular, in landscapes where human land-use is intense, and low traffic volumes, minor roads often provide critical habitat and refuge for many native species (Spooner and Smallbone, 2009). Nevertheless, although extensive research has been carried out, few such studies exist which examine the impact of roadsides on biodiversity under an intensive and complex human land-use system in mountain landscapes. Hence, there is little knowledge of the role of this kind of linear infrastructure on plant species conservation in the mountain landscape compared to other land uses. Moreover, in the southern African region, despite its growing road infrastructure and other linear infrastructure (World Bank, 2018), there are few studies available in the international literature about the effects of roads on biodiversity (Laurance et al., 2009; Bennett, 2017; Lázaro-Lobo and Ervin, 2019).
While some research has studied land-use's impact on tree species diversity, few studies have attempted to investigate the effects of roads and land use on plant species diversity, rarely assessing more than one plant life form. As a result, there is much less information concerning how land-use change affects plant species (e.g., trees, shrubs, herbaceous, and grass) in mountain landscapes. However, measures of species richness of different plant life forms have several benefits: increases the efficiency and stability of some ecosystem functions (Tilman, 1996; Peterson et al., 1998), provide information on susceptibility to invasions and trophic structure necessary for ecosystem resilience (Ruiz-Jaen and Aide, 2005), and benefits for agricultural production and livestock (Tilman et al., 2001; Gaujour et al., 2012) as well as make up a source of food for animals and humans.
This paper aims to assess the role of roads on plant species diversity compared to other land-use types in the mountain landscape of southern Africa. The specific objectives of this paper were to investigate: (1) the variation pattern of species richness, species diversity and species composition of four plant life forms (regenerated trees, shrub, herbaceous, and grass species) amongst land-uses including roads; (2) the beta-diversity of the four-plant life forms amongst land-uses and roads. This research will hopefully contribute to a deeper understanding of how land use and roads impact and shape different plant species diversity in the mountain landscape in Mozambique. First, this paper describes a range of alpha-diversity measures, then advances to beta diversity among land-uses and roads.
Methods
Study Area
We carried out this study at Moribane Forest Reserve (MFR), in Eastern Chimanimani Mountain range, located within the Dombe Administrative Post of Sussundenga district, Manica province in central Mozambique (19° 45′S, 33° 22′ E) (Figure 1). The area of MFR is about 53 km2, and was proclaimed a conservation area in 1957 (De Sousa, 1968). Since 2000, the MFR has been part of the Chimanimani National Park (a national park in June 2020), a Transfrontier Conservation Area between Mozambique and Zimbabwe. The climate is classified as tropical, modified by altitude, according to Köppen climate classification (Seabra, 1961). The altitude range is 200–690 m a.s.l., and the mean annual temperature range is 17–24°C. The mean annual rainfall range is 1,200–1,400 mm (Dutton and Dutton, 1973), and the soils range from sandy loam to sandy clay (Seabra, 1961). The MFR supports a mosaic of several woodlands and forest types co-dominated by deciduous tree species, such as Newtonia buchananii (Baker) Gilbert & Boutique (Leguminosae), Pteleopsis myrtifolia (M. A. Lawson) Engl. & Diels (Combretaceae), Millettia stuhlmannii Taub. (Leguminosae), Albizia gummifera (J. F. Gmel.) C. A. Sm. (Leguminosae), Khaya anthotheca (Welw.) C. DC., among others (Müller et al., 2005; Monteiro et al., 2011).
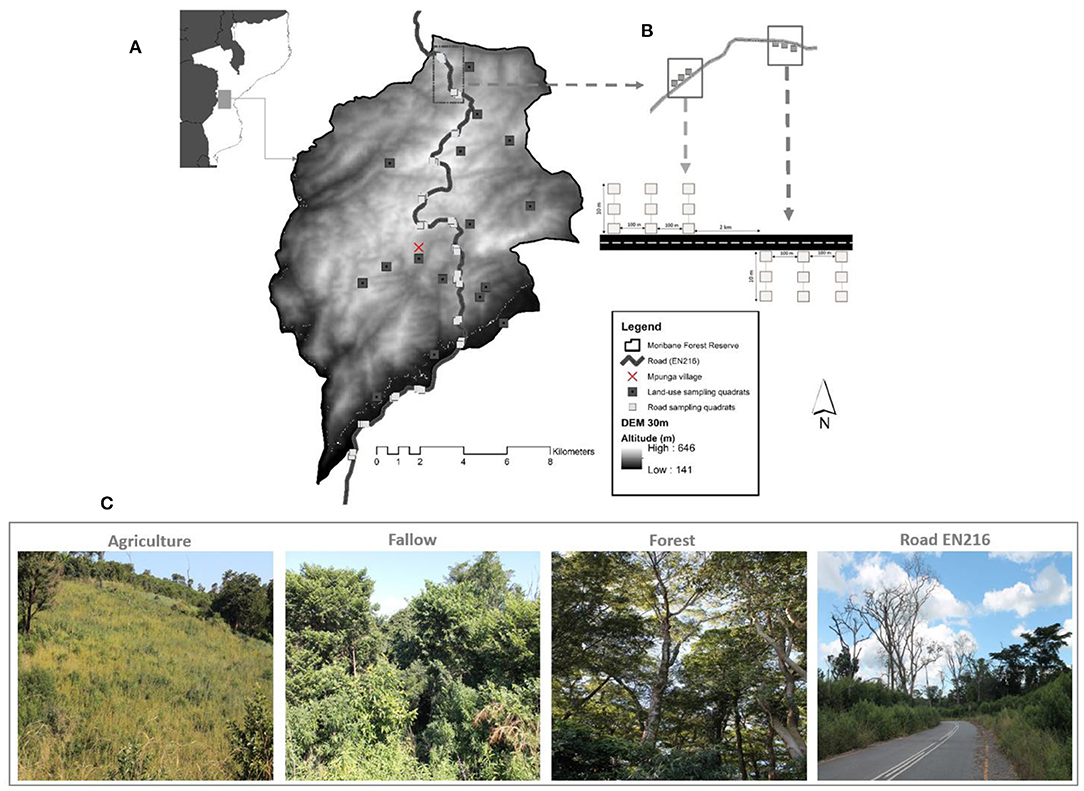
Figure 1. Moribane Forest Reserve, Sussundenga district, Manica province with the distribution of land-use sampling quadrats and road sampling quadrats along paved road EN 216; the background map shows the national location of MFR in Mozambique (A). The sets of 3 transects for each side of the Road was 2 km apart, and the three transects contained three quadrants 5 m apart (B). Land use types targeted in this study in MFR (C). Photos by Sá N. Lisboa.
The main National Road (EN 216) crosses the forest reserve, is 230 km long and connects the district capital of Sussundenga to the capital city of Chimoio, to the Administrative Post at Dombe to the town of Espungabera (capital of Mossurize district), and eventually to the border of Zimbabwe, in Manica province. The gravel road was constructed in colonial times and rehabilitated and paved in 2014, overcoming high hills and sloppy terrain. The road's highest peak reached 690 m at the northern edge of the reserve and the lowest with 102 m at the south-east. The objective of the road is to improve the circulation and goods along the Road. Road EN216 is one of the busiest in Manica province and is a route for domestic traffic, transports goods from neighboring countries, particularly Zimbabwe and South Africa, from the border at Espungabera, in Mozambique and Mount Slinda in Zimbabwe (Macauhub, 2010). The roadside verge is about 10–12 m wide with cut and fills slopes. Agricultural fields, forests, and woodlands occupy the road from the verge to outwards. The primary land-cover type is the moist evergreen forest (Müller et al., 2005) on the western side of the road, which lies in the core area of the forest reserve with 291 ± 141 Mg ha−1 (mean ± 95% confidence level) of above-ground biomass (Lisboa et al., 2018). There are intermixtures of shifting agriculture on the eastern side of the road, mainly banana and intercropping with maise, cassava, and other subsistence crops, fallow land, and rural settlement.
Data Collection
In mid-May of 2019, at the onset of the wet season in Mozambique, a study was conducted to assess the roadside influence on plant diversity in Moribane Forest Reserve. Excluding roadside—within 12 m from the road edge; the considered land-use types or categories are (i) agriculture (cropped areas), (ii) fallow land (abandoned agriculture land in regeneration), (iii), and forest (pristine forest). The sampling strategy of plant species of road assemblage differed according to land-uses. Roads are linear structures that cut through several other uses or land cover. Therefore, our focus was on the nested effects of roads within different land-use types. The experimental design we applied singled out the impact of roads and allowed us to compare plant communities under different use regimes. We established three 10 m transects in one direction (East-West) perpendicular to the road with 100 m spacing between them measured along the road. The sets of the three transects were established alternating roadsides in every 2 km linear distance yielding 22 transects on one side and 23 on another side (n = 45 transects) (Figure 1). Within each 10 m transect sample sites, three points from the road edge outwards were selected (0, 5, and 10 m). We chose the 10 m maximum distance as this buffer was deemed the maximum road verge distance with high slopes and hills.
The agricultural lands or slash and burn agriculture (hereafter, Agriculture), slash and burn fallow (hereafter, Fallow), and undisturbed forests (hereafter, Forest) were detected combining land-use maps from 2018 (Eriksson, 2020). In addition, we combined the aerial drone images from 2019 and household interviews with local farmers identified by contacting traditional leaders to determine the Fallow land and Agriculture fields relying on their local knowledge. We then randomly established 24 quadrats in the Agriculture plots and Fallow and 26 in the undisturbed Forest. The sampled fields were active agriculture plots with crops on the ground. In contrast, the sampled Fallow land included abandoned agricultural areas subjected to slash-and-burn practices, as a regeneration process, between 3 and 5 years earlier. Thus, the sample was representative concerning the primary purpose of this study, on comparing the species diversity of roadside against other land-uses.
In all land-use plots (Agriculture, Fallow, Forest) and Road, we sampled grass species within 1 × 1m quadrats and 2 × 2 m quadrats for non-grass species (regenerated trees, regenerated shrubs, and herbaceous). A botanist identified the presence and name of all plant species. From all plant species that the botanist could not identify with certainty in the field, voucher specimens were collected, pressed, and brought to the Herbarium of Eduardo Mondlane University in Maputo for identification. We used several species identification keys to identify the botanical name of regenerated trees and shrub species (van Wyk and van Wyk, 1997; Coates Palgrave, 2003), herbaceous and grass species (Pooley and Carruthers, 1999; van Oudtshoorn, 2012). Plants from non-grass quadrats we categorised as regenerated tree species (hereafter, tree species), regenerated shrub species (hereafter, shrub species), and herbaceous species. All plant species were classified as exotic or indigenous using Tree of Southern Africa (Coates Palgrave, 2003). We also used the Invasive species compendium (CABI, 2020) to classify the invasiveness of each species.
Statistical Analyses
Alpha Diversity
We computed the species diversity using an unbiased non-parametric estimator of the Shannon and Simpson diversity indices (Chao and Shen, 2003). We used Hill numbers or the effective numbers of species to quantify the species diversity through the Shannon index and Simpson index (Hill, 1973; Jost, 2006, 2007). The parameter q controls the sensitivity of the measure to species relative abundance (Table 1), that is, the influence that rare, typical, or dominant species may have in the estimation of diversity (Jost, 2006; Moreno et al., 2011; Gotelli and Chao, 2013; Rendón-Sandoval et al., 2020). When q = 0 (0D, diversity of order 0), the abundance of species does not influence the value of q, thus providing disproportionate weight to rare species, and the obtained value is equivalent to the species richness. When q = 1 (1D, diversity of order 1), all species have a weight proportional to their abundance in the community (therefore, one of the best parameters to estimate diversity), and it is equivalent to the exponential of Shannon's entropy index calculated with the natural logarithm (1D = exp H'). Therefore, the Hill number of order 1 is the number of typical species in the community. When q = 2 (2D, diversity of order 2), the abundant species have a more substantial influence, and rare species are discounted; hence, this diversity can be interpreted as the number of dominant species in the community and is equivalent to the inverse value of Simpson's dominance index (2D = 1/D). Table 1 presents formulas used in this study of species richness index.
Rarefaction and Extrapolation
Rarefaction is a statistical interpolation method of rarefying or thinning a reference sample by drawing random subsets of individuals (or samples) to standardize the comparison of biological diversity based on a specific number of individuals (Gotelli and Chao, 2013). We constructed sample-and coverage-based interpolation and extrapolation curves to determine how the species diversity of tree, shrub, herbaceous, and grass increased with increasing sampling effort and completeness for each land-use. We used incidence data for interpolation and extrapolation of species richness, the effective number of Shannon diversity, and Simpson diversity. The integrated curves based on sampling theory that smoothly link rarefaction and prediction standardise samples based on sample size or sample completeness and facilitate the comparison of biodiversity data for more extensive and smaller samples (Chao et al., 2014). Coverage-based curves were plotted against rarefied sample completeness to illustrate diversity estimates with sample coverage (Hsieh et al., 2016). We applied 100 bootstrap replicates to estimate 95% confidence intervals. We used the 95% confidence intervals to determine if differences in plant diversity among land-uses were statistically significant. Non-overlapping at 95% confidence intervals, we considered indicating definite significant differences at 5% risk-of-error level either rarefied or extrapolated curves (Chao et al., 2014).
Beta Diversity
We assessed a beta-diversity or (dis)similarities in each plant life form among land-uses for every pair of land-use using the Sorensen index for presence-absence data following the Baselga and Orme (2012). Beta diversity is partitioned into dissimilarity due to species replacement and dissimilarity due to nestedness (Baselga, 2010). Here we computed total dissimilarity (βSOR), as well as the respective turnover (βSIM) and nestedness (βSNE) components (Baselga, 2010; Baselga and Orme, 2012) for each plant life form. Species turnover implies the simultaneous gain and loss of species due to environmental filtering, competition, and historical events (Leprieur et al., 2011; Legendre, 2014). In contrast, nestedness is a richness difference pattern characterized by the species being a strict subset of the species at a more prosperous site in terms of species (Legendre, 2014). For multivariate community assemblage analyses, we applied the Constrained Analysis of Principal Coordinates (CAP) method on the Bray-Curtis dissimilarity matrix, following the protocol in Anderson and Willis (2003). Anderson and Willis advocate using the number of principal coordinate axes that result in the best prediction of group identities of the sites. We applied the non-parametric multivariate analysis of variance (NPMANOVA) to test the differences between plant life forms and land-use types. We first computed the unconstrained ordination analysis to examine the overall patterns across the entire data cloud and any differences in within-group variability or spread. The first step was to choose the number of principal coordinate (PCO) axes (m) to include in the CAP analysis. The PCO we did by plotting the proportion of correct allocations obtained with increases in the number of axes included in the analysis. We used functions available in the packages MASS (Venables and Ripley, 2002), iNEXT (Hsieh et al., 2016), betapart (Baselga et al., 2018), vegan (Oksanen et al., 2019) and BiodiversityR (Kindt, 2019) to compute alpha and beta diversity using R environment (R Core Team, 2018).
Results
Plant Species Composition in All Assemblage
We identified 216 plant species of different plant life forms, and we observed the presence and abundance of all these species within all sampling quadrats. Figure 2 shows the total numbers of species recorded of each plant life form. We recorded 43 trees species belonging to 22 families, mainly of Fabaceae and Euphorbiaceae, 51 shrub species belonging to 26 families with Asteraceae, Fabaceae, Verbenaceae, and Apocynaceae as the families with most species recorded. Herbaceous had the highest number of species, with 84 species belonging to 34 families with five most registered families (Malvaceae, Asteraceae, Lamiaceae, Fabaceae, and Rubiaceae). In addition, we detected 34 grass species of the Poaceae family. The list of species and families to which the species belong is in Supplementary Table 1. We recorded a few trees' species (Albizia adianthifolia, Millettia stuhlmannii, and Steganotaenia araliaceae) that we found in all land-use types with high areal coverage in the Forest interior. The shrub species such as Vernonanthura phosphorica, Mucuna coriacia, Lantana camara are the most common in the study area. The herbaceous species sampled in the roadside and other land-uses are Bidens pilosa, Commelina benghalensis, Ipomoea sp., and Sida alba. We found more than 70% of grass species thriving on the roadside. Therefore, we only recorded four grass species (Eleusine indica, Panicum deustum, Panicum maximum, and Panicum heterostachyum) recorded in all land-use types, but with sporadic presence in the Fallow and Forest interior.
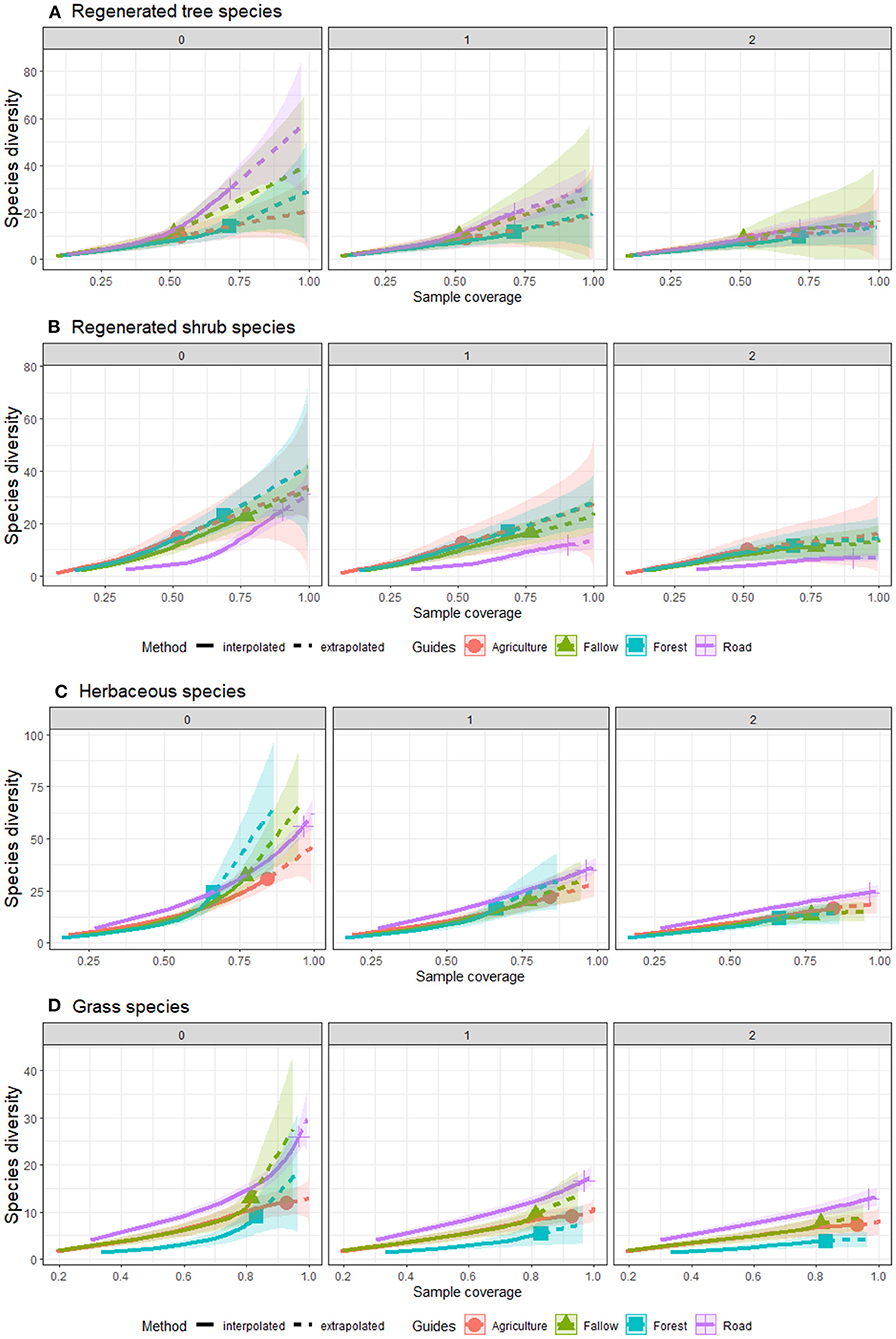
Figure 2. Comparison of sample coverage-based interpolation and extrapolation for species richness (q = 0), Shannon index (q = 1) and Simpson index (q = 2), across four land-uses for (A) tree, (B) shrub, (C) herbaceous, and (D) grass in Moribane Forest Reserve, central Mozambique.
Roadside had the highest recordings of invasive species amongst land-uses, but similar to Agriculture. We recorded around 101 invasive species in the whole assemblage, 31 on the roadside, and 70 from another land-use type with lower invasiveness in the Agriculture fields. We recorded 41 tree species in the entire assemblage, and 12% are invasive species; for shrubs, we found 51 species with 30% registered as invasive species. We recorded 83 herbaceous species, and 31% are invasive, whereas for grass species, from 34 total species, 58% were invasive (14 species), and the invasiveness of nine species is unknown. Grass species had the highest number of invasive species, following herbaceous and shrubs and lastly trees species. Roadside had the most considerable number of invasive species of all plant life forms with 31 alien species, following Agriculture with 30, Fallow with 20, and Forest with 19 invasive species. Vernonanthura phosphorica, a shrub species, was the most common and dominant invasive species in the study area and most recorded in the roadside (65%), following Fallow (15%), Forest (13%), and Agriculture (7%). Plectranthus fruticosus and Alysicarpus vaginalis were the most common invasive species from herbaceous, and Melinis repens from grasses.
Plant Species Diversity Among Land-Use Types
Table 2 shows the observed and estimated species diversity of the plant life forms recorded in Moribane Forest Reserve for roadside and other land uses. It is apparent from this table that roadside had the highest observed species richness (0D) with 30 trees, 25 shrubs, 56 herbaceous, and 26 grass species significantly, and had a high observed effective number of species (1D e 2D) of the tree, herbaceous, and grass species against other land-uses. Interestingly, roadside holds more tree species than Forest with 30 observed and 59 estimated tree species against 14 observed and 29 estimated for Forest. However, there were no significant difference in observed species richness and the effective number of species between roadside and other land uses for shrub species. Moreover, instead of observed numbers, the roadside also had the highest estimated or extrapolated species richness values of tree, herbaceous, and grass species, although statistically equal to the Fallow. However, the effective number of herbaceous and grass species was significantly higher on the roadside than within the other three land-uses. Forest had particularly higher observed richness of trees (14 sp.), shrubs (23 sp.), and herbaceous (24 sp.) than Agriculture with ten trees, 15 shrubs, and 31 herbaceous species; but with no significant difference with Fallow with 11 trees and 23 shrubs. Agriculture and Fallow, however, had higher observed or estimated grass species richness and the effective number of grass species than Forest.
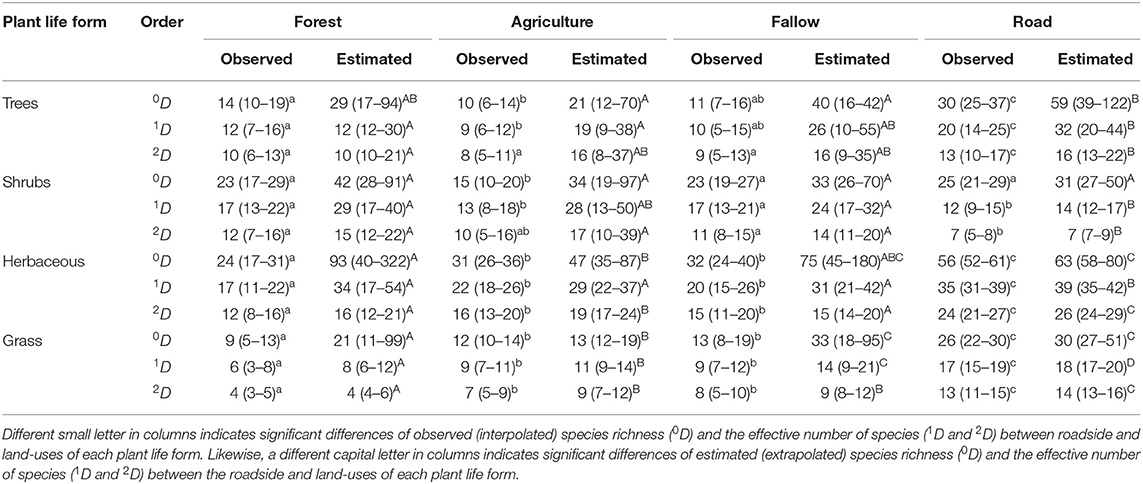
Table 2. Comparison of sample-based interpolation [Observed (CI at 95%)] and extrapolation [Estimated (CI at 95%)] for species richness (0D), the effective number of species from Shannon (1D), and effective number from Simpson (2D), of roadside against land-uses for each plant life form in Moribane Forest Reserve, Mozambique.
There were significant differences between the observed species number and estimated species number in all land uses and plant life forms, mainly for tree species (Table 2). The estimated richness of tree species was not significantly different between Forest and Agriculture and Fallow and Road. Fallow had high differences between observed and estimated or extrapolated tree species richness, whereas roadside had the lowest differences recorded among land uses. The estimated number of shrub species was not significantly different amongst land-uses ranging from 31 recorded on the roadside to 42 species recorded in Forest. The differences between the observed and estimated number of herbaceous and grass species were prominent in the Forest following Fallow, Agriculture and roadside. The estimated number of herbaceous species in the Fallow were not significantly different from other land uses, including roadside.
In contrast, the Agriculture assemblage had the lowest estimated number (n = 47 sp.) of herbaceous species among land uses, and Forest had the the highest number of estimated species (n = 93 sp.) following Fallow (n = 75 sp.). In addition, agriculture had the lowest number of estimated grasses species with 13 species, following Forest with 21 species. Roadside and Fallow had more estimated richness species with 30 and 33 species, respectively.
Sampling Coverage and Species Diversity of Plant Life Form Among Land Uses
Our results suggest that species diversity increases with sample coverage (Figure 2). This trend is particularly evident in the species richness (q = 0) rather than the effective number of species (Shannon, q = 1) and (Simpson, q = 2). For instance, tree species revealed that richness would increase with higher sample coverage on the roadside. On the other hand, the number of significant differences for Shannon and Simpson diversity indices did not increase with increased sample coverage among land use. Furthermore, Forest revealed that it would not significantly increase its tree species diversity if we expanded the sample coverage. Whereas, for shrubs species, Agriculture, Fallow and Forest indicate a significant increase of species diversity when increasing the sample coverage, even though the extrapolated curve (dashed line) had a low slope and did not increase much. The roadside had a lower species diversity even when growing the sample coverage. These results suggest that roadside and Fallow have fewer species of shrubs, but with high tree species diversity among land uses.
Agriculture and Fallow had the same kinds of the trend of species diversity that increased when increasing the sample coverage either with interpolation or extrapolation for grass species (Figure 2). In contrast, roadside and Forest had significantly different trends compared to each other. Roadside markedly suggests a high increase of grass species diversity with increasing sample coverage. In contrast, Forest revealed that the species richness would increase if the sample coverage increased, but the species diversity (Shannon and Simpson) showed a moderate increase. Herbaceous species showed the richness of roadside risen markedly in the interpolation data; in the extrapolation, the richness of species richness become lower than Forest and Fallow.
The roadside achieved the sample coverage of all plant life forms with interpolation (Figure 3). This result suggests that we established enough sample units on the roadside to sample the herbaceous and grass species fully. Shannon's and Simpson's effective number of species became asymptotic before extrapolation. Agriculture fields revealed a high sample coverage in all plant life forms except for regenerated tree species, whereas Forest showed a sloped sample coverage curve. The sample coverage curve of Fallow was slightly sloped for shrub and herbaceous species. The interpolated number of samples (quadrats) was lower to address the tree species in all land-use types except on the roadside. However, the extrapolation suggests that all land-use types, except roadside, would need at least 100 sample units to cover the species diversity in each assemblage. Roadside is the only land-use type that reached a sufficient sample coverage either with interpolation or extrapolation for all plant life forms.
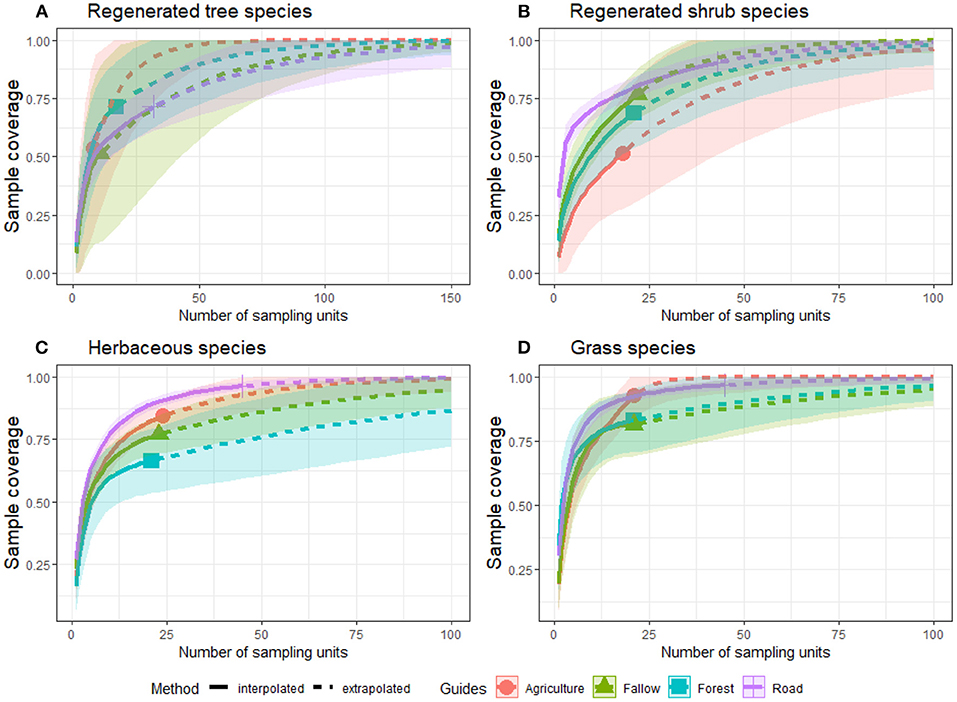
Figure 3. Sample-based interpolation and extrapolation for sample coverage compared to plant life forms (A–D) across land-uses in Moribane Forest Reserve, central Mozambique.
Beta-Diversity and Dissimilarity of Plant Life Form Among Land-Uses
The first two PCO axes explained 80.17% and 15.17% of the variability in the original dissimilarity matrix for tree species. For shrubs, the first two PCO axes explained about 90.74 and 5.72%, and for herbaceous, the proportions were 81.54% and 12.43% for the first two PCO axes. Finally, for grass species, the first two PCO axes explained 87.50 and 10.42% of the variability in the original dissimilarity matrix. The non-parametric multivariate analysis of variance indicated no overlaps among plant life form across land-uses, suggesting high species dissimilarity of shrub (F = 5.50, P < 0.001), herbaceous (F = 5.75, P < 0.001) and grass (F = 4.70, P < 0.001) species. Meanwhile, although tree species showed a significant dissimilarity (F = 2.43, P < 0.001) amongst land-uses, the scatter plot of points (Figure 4) suggested a great overlap among groups, which means that the scatter plot of points. Interestingly, roadside plots had unshared shrubs, herbaceous, and grass species with other land-uses (Figure 4). Herbaceous are the only plant life form with high dissimilarity amongst almost all land-uses, suggesting a great difference among groups.
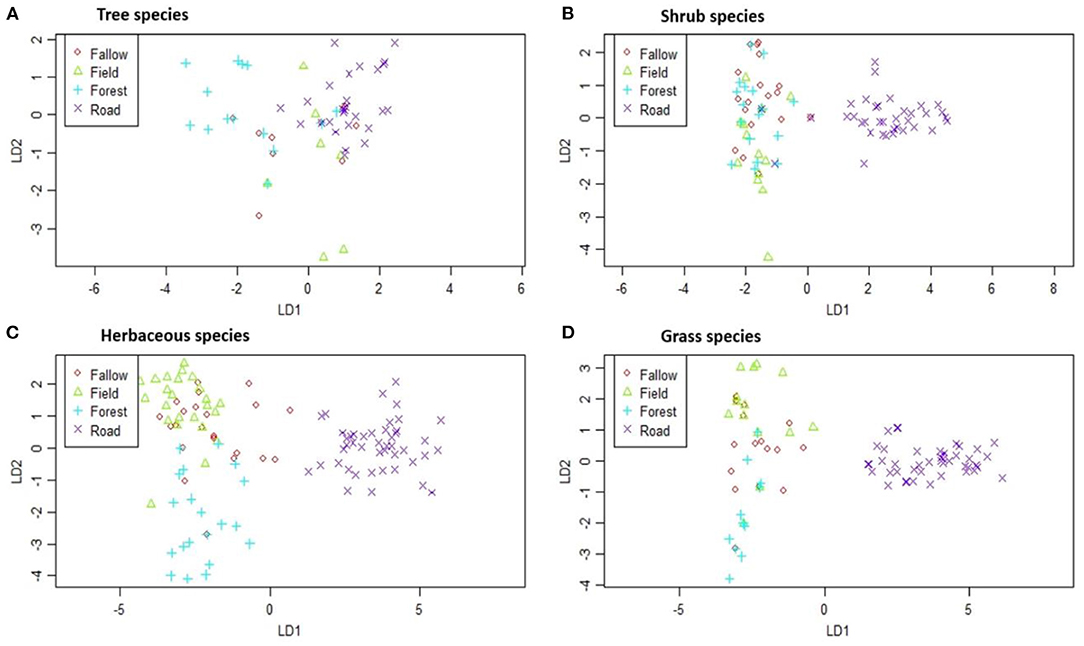
Figure 4. Dissimilarity of (A) tree species, (B) shrub species, (C) herbaceous species, and (D) grass species among land-use types in Moribane Forest Reserve, central Mozambique. The symbols represent each plant life form species in each land-use type.
Most of the species are specific to the roadside: we recorded a total of 43 tree species on the roadside, and 49% are particular to this assemblage; we recorded a total of 51 shrub species in the roadside, and 21% of them are specific to this assemblage; we registered a total of 84 herbaceous species, and 36% of them are particular to the roadside; and of the 34 recorded grass species, 41% are specific to this assemblage. The results revealed that there are four trees (10% of total species), nine shrubs (18%), 18 herbaceous (23%) and eight (24%) grass species shared between Agriculture and Fallow. We found five trees, four shrubs, seven herbaceous, and three grass species-specific to the Agriculture; and three trees, four shrubs, six herbaceous and two grass species-specific to the Fallow.
The five most recorded species-specific to the roadside for tree species were: Senna sp., Pterocarpus rotundifolius, Albizia versicolor, Dalbergia sp., and Celtis gomphophylla; for shrubs, species were Mucuna coriacia, Deinbollia sp., Aspilia mossambicensis, Indigofera woodii, and Abutilon angulatum. The five most common herbaceous species were Vernonia poskeana, Asystasia sp., Alysicarpus vaginalis, Vernonia glabra and Oldenlandia diffusa; and grass species were Cymbopogon winterianus, Cyperus sp., Digitaria sp., Eragrotis sp., and Heteropogon contortus.
The estimated overall beta diversity of tree species (βSOR = 0.75) is similar to the diversity of shrub species (βSOR = 0.70). The high similarity for tree species and shrubs species amongst land-uses means that these species can be found in all land uses. In contrast, the grass species had the lowest overall beta diversity (βSOR = 0.65), similar to the herbaceous species' value (βSOR = 0.68). However, when this overall beta diversity is partitioned into its turnover and nestedness components, it remains clear that processes underlying these beta-diversity values are different. For tree species (βSIM = 0.60, βSNE = 0.15), shrub species (βSIM = 0.65, βSNE = 0.05) and herbaceous species (βSIM = 0.56, βSNE = 0.12) their multiple beta-diversity patterns are almost completely caused by species replacement between land-uses. The overall beta diversity for grass species mainly results from species replacement (βSIM = 0.45) and slightly by species loss (βSNE = 0.20) from roadside toward the Forest. In other words, the dissimilarity of land-uses is due to the effect of turnover instead of nestedness patterns. In Figure 4, broadly, what stands out is that the roadside had high similarity with the Forest within all plant life forms. In contrast, tree species had the highest similarity between roadside and Agriculture (βSOR = 0.75) and roadside and Fallow (βSOR = 0.76).
Discussion
Alpha-Diversity of Roadside and Land-Uses of Different Plant Life Form
This study addressed the impact of land use, including roads, on alpha and beta-diversity in the mountain landscape of Moribane Forest Reserve, central Mozambique. To our knowledge, this is the first study in southern Africa that combines roads and other land-uses to assess its effect on the diversity of different plant life forms in the mountain landscape. We found evidence for the positive impact of the roadside on species richness and species diversity. However, this should not be surprising since the broader literature suggests that anthropogenic gaps such as roadsides can increase plant abundance and diversity (Suárez-Esteban et al., 2016; Root-Bernstein and Svenning, 2018; Lázaro-Lobo and Ervin, 2019). The main reasons for the presence of different species, including a high proportion of alien species along the roadside, are often related to changes in physical and chemical properties of soil (Ullmann et al., 1995), light conditions (Parendes and Jones, 2000; Gálhidy et al., 2006; Delgado et al., 2007), as well as microclimate (Pauchard and Alaback, 2004). This condition allows the invasive species to thrive in the disturbed site and increase the species diversity. As a result, we registered more invasive species in the most disturbed areas such as roadside, Agriculture and Fallow. Another plausible explanation of the current findings is tree species seed banks (Devlaeminck et al., 2005). The seed bank of the study area seems to hold more light tolerant species that grow together with invasive species in the disturbed sites.
Roadside had the highest sample coverage concerning other land-uses because the sampling effort was higher in this assemblage. However, this issue was not very problematic as using estimated numbers of species rather than actual sampled numbers of species mitigated the effects of varying sampling efforts amongst land-uses. Meanwhile, it provided a suitable sample size effort that might be adopted in future studies (Rendón-Sandoval et al., 2020). Furthermore, the roadside had the highest native species diversity of each plant life form. Therefore, we assumed that this species could thrive and survive the adverse new conditions created by road construction (Fernandes et al., 2018). Furthermore, Ervin (2009) argues that some native species adapt to disturbed environments and expand their distributional ranges along roadsides.
The richness of herbaceous and grasses species was significantly lower in the Forest interior. These finds may be due to the abiotic conditions in the forest interior, especially the low light level and the thick litter layer, unfavorable for germination and establishment of these shade-intolerant species (Goldblum, 1997; Parendes and Jones, 2000; Devlaeminck et al., 2005). On the other hand, the higher species richness and diversity of herbaceous, grass, and shrub species recorded on the roadside, Fallow, and Agriculture fields can be due to the rising gap area. This finding broadly supports the work of other studies in this area, linking species diversity with gaps size (Goldblum, 1997; Anderson and Leopold, 2002; Gálhidy et al., 2006; Naaf and Wulf, 2007; Vajari et al., 2011).
Fallow and Agriculture fields did not show significant differences in species richness and diversity of herbaceous and grass species. This result means that the individual distribution of all herbaceous and grass species was homogeneous in Fallow and Agriculture. Agricultural fields in the study area shift cultivation characterized by temporary, periodic, or intermittent. For instance, a plot of land is usually cultivated 8–10 years with maze and beans and then replaced with crops that need lower soil fertility demanding such as sorgo for a short time, usually 4–5 years (Aweto, 2013). After that, the land is left uncultivated and allowed to revert to Fallow or 'bush' to restore soil fertility through vegetation colonization and development (Aweto, 2013). Therefore, the same land management is a plausible explanation for why the species richness and diversity were not statistically different between Fallow and Agriculture.
One anticipated finding was that roadside had the highest number of invasive species amongst land-uses agreeing with the hypothesis of the impact of roadside on invasive species spreading. It suggested that vehicle traffic facilitates invasive non-native plant species (Parendes and Jones, 2000; Hansen and Clevenger, 2005; Follak et al., 2018). This reason appears to be the case because the Road (EN 216) lies inland, with reasonably little traffic explaining the increase in alien and native indigenous species. For example, Vernonanthura phosphorica is the most common invasive shrub species in the study area, with higher recording in the roadside and Fallow. However, the species were also sporadically observed in forest and woodland interiors lowland areas. These findings raise questions regarding the nature and extent of V. phosphorica in the study area. This species was introduced as a bee-fodder plant in the 90s and is currently taking over lowland forest, and woodland cleared, burnt, and left to rest (Timberlake, 2017). V. phosphorica is growing in the roadside edge and has high canopy cover and high litter cover, allowing less light to reach the soil, favoring non-native species to thrive. This species flourish under high soil temperature, nitrogen and phosphorus content, and low soil pH and carbon (Ngarakana and Kativu, 2017). Since a substantial impact on the community scale is associated with reducing species diversity at higher rankings, invaders with a high impact represent a severe hazard to the landscape (Hejda et al., 2009). Given that this species is spreading faster in the study area, reaching mountain areas above 1,000 m altitude (Timberlake, 2017), a management decision is urgent to control the invasiveness of this species. Furthermore, biologists have known the Chimanimani Mountain landscape as an area of exceptional biodiversity with several plant species that are endemics make the region unique in Mozambique (Timberlake, 2017). Therefore, conservationists should undertake further research to investigate the negative effect of V. phosphorica on community species composition in the Chimanimani Mountain landscape.
Beta-Diversity Assessment of Multiple and Pairwise Comparisons
According to the CAP analysis, there were a lot of tree species common to all land-use types, which caused the point clouds in Figure 4 to overlap partly. In contrast, other plant life forms were mainly specific to the roadside. These finds revealed that the roadside consists primarily of pioneer and light-demanding plant species (Monteiro et al., 2011; Arroyo-Rodríguez et al., 2013). In the current study, comparing dissimilarity between roadside and other land-uses showed that the roadside was more similar to the Forest for tree species and significantly different from other land uses. This result can be due to the road edge effects that sometimes favor tree species because their seedlings need light availability and exposed soil (Lázaro-Lobo and Ervin, 2019). However, a further study on assessing the tree species dispersal and establishment strategy across land-use types suggests information concerning the seed banks and abiotic environment (e.g., soil type or light conditions).
Our data showed a high beta-diversity among sites for tree species (75%), shrubs (70%), herbaceous (68%), and grass (65%), reflected mainly by high species turnover (replacement) rather than nestedness (differences in species richness). Species turnover demonstrates the replacement of species from one site to the next. It may result from either species gain or loss due to environmental sorting, historical constraints, and negative interaction (Baselga, 2010). Moreover, species turnover has been proposed to reflect deterministic processes, such as species' adaptations to the environmental gradient. It can result from limited dispersal coupled with speciation, delayed response to climatic change, or other historical effects (Condit et al., 2002). Thus, the local environmental variables might be the main drivers of total beta diversity, nestedness, and turnover (Hill et al., 2017). However, further studies, which take these variables into account, will need to be undertaken.
Within heterogeneous landscapes, species can track suitable environmental gradients where dispersal is sufficient, increasing species turnover. Gianuca et al. (2017) stressed that, in homogeneous landscapes, increased scattering has decreased species turnover resulting in assemblages that are nested subsets of those sites with higher species richness. Therefore, according to this statement, it is thought that the land use in the study area is heterogeneous, increasing the species replacement in the study area.
Conclusion
We designed this study to assess the impacts of land use on alpha and beta-diversity of different plant life forms in the Chimanimani Mountain landscape in Mozambique. The most prominent finding from this study is that roadside had the highest species richness and species diversity compared to other land-use types. However, the small sample size from Agriculture, Fallow, and Forest did not compare reasonably with the observed species diversity. Notwithstanding these limitations, the study suggests that the extrapolated species diversity has mitigated the effect of the sampling effort. The conclusions of this study make several contributions to the current literature. Firstly, taken together, this result suggests that analysis that includes roads as a land-use type provides a more quantifiable measure of the impact of land use on biodiversity in the conservation area where roads and other anthropogenic land use are evident. Secondly, roads can play a significant role in species conservation as linear ecosystems in our study area, causing likely a trade-off of tree species between roadside and land-uses (i.e., agriculture). Lastly, this study exposes and promotes the need to explore road ecology more, a developing field in southern Africa. This study contributes toward a better understanding of the roads' impact on species diversity compared with southern Africa's most dominant land-use systems. However, further studies countrywide regarding the role of different types of roads and land use in species conservation will be worthwhile.
Data Availability Statement
The original contributions presented in the study are included in the article/Supplementary Material, further inquiries can be directed to the corresponding author.
Author Contributions
SL was responsible for original draft preparation, formal statistical analysis, revised the manuscript, and submitted the article. SL, FD, and AS conducted field data collection. EM performed field data collection and species identification. EV, AS, AL, and SL research conceptualization and conceived the study. AS, EV, and AL funding acquisition. EV, AS, AL, and FD writing—review and editing. All authors have read and agreed to the published version of the manuscript.
Funding
This work (SuMNature project, Number 21000038041) was funded with Finland's Ministry for Foreign Affairs support.
Conflict of Interest
The authors declare that the research was conducted in the absence of any commercial or financial relationships that could be construed as a potential conflict of interest.
Publisher's Note
All claims expressed in this article are solely those of the authors and do not necessarily represent those of their affiliated organizations, or those of the publisher, the editors and the reviewers. Any product that may be evaluated in this article, or claim that may be made by its manufacturer, is not guaranteed or endorsed by the publisher.
Acknowledgments
Department of Forestry Engineering from Eduardo Mondlane University in Mozambique for paying the article processing charges. The co-authors wish to thank the University Zambeze SuMNature team (Gonzaga Chilole, Roberto Chuquela) for their assistance with field research. The first authors also want to thank the University of Jyvaskyla SuMNature team (Prof. Pekka Virtanen, Dr. Elisa Vallius, and Dr. Anssi Lensu) for their assistance and collaboration at the Department of Environmental Science in Finland. We also thank the reviewers for their valuable suggestions and comments.
Supplementary Material
The Supplementary Material for this article can be found online at: https://www.frontiersin.org/articles/10.3389/fcosc.2022.829690/full#supplementary-material
References
Alamgir, M., Campbell, M. J., Sloan, S., Goosem, M., Clements, G. R., Mahmoud, M. I., et al. (2017). Economic, socio-political and environmental risks of road development in the tropics. Curr. Biol. 27, R1130–R1140. doi: 10.1016/j.cub.2017.08.067
Anderson, K. L., and Leopold, D. J. (2002). The role of canopy gaps in maintaining vascular plant diversity at a forested wetland in New York state. J. Torrey Bot. Soc. 129, 238–250. doi: 10.2307/3088774
Anderson, M. J., and Willis, T. J. (2003). Canonical analysis of principal coordinates: A useful method of constrained ordination for ecology. Ecology 84, 511–525. doi: 10.1890/0012-9658(2003)084[0511:CAOPCA]2.0.CO;2
Arroyo-Rodríguez, V., Rös, M., Escobar, F., Melo, F. P. L., Santos, B. A., Tabarelli, M., et al. (2013). Plant β-diversity in fragmented rain forests: Testing floristic homogenization and differentiation hypotheses. J. Ecol. 101, 1449–1458. doi: 10.1111/1365-2745.12153
Aweto, A. O.. (2013). Shifting cultivation and secondary succession in the tropics. CABI. 209. doi: 10.1079/9781780640434.0000
Barber, C. P., Cochrane, M. A., Souza, C. M., and Laurance, W. F. (2014). Roads, deforestation, and the mitigating effect of protected areas in the Amazon. Biol. Conserv. 177, 203–209. doi: 10.1016/j.biocon.2014.07.004
Baselga, A.. (2010). Partitioning the turnover and nestedness components of beta diversity. Glob. Ecol. Biogeography 19, 134–143. doi: 10.1111/j.1466-8238.2009.00490.x
Baselga, A., and Orme, C. D. L. (2012). Betapart: An R package for the study of beta diversity. Methods Ecol Evol. 3, 808–812. doi: 10.1111/j.2041-210X.2012.00224.x
Baselga, A., Orme, D., Villeger, S., De Bortoli, J., Leprieur, F., Logez, M., et al. (2018). Partitioning beta diversity into turnover and nestedness components. Cran 2018, 1–42.
Bennett, V. J.. (2017). Effects of road density and pattern on the conservation of species and biodiversity. Curr. Landscape Ecol. Rep. 2, 1–11. doi: 10.1007/s40823-017-0020-6
Biggs, R., Simons, H., Bakkenes, M., Scholes, R. J., Eickhout, B., van Vuuren, D., et al. (2008). Scenarios of biodiversity loss in southern Africa in the 21st century. Glob. Environ. Change 18, 296–309. doi: 10.1016/j.gloenvcha.2008.02.001
Chao, A., Chiu, C. H., and Jost, L. (2014). Unifying species diversity, phylogenetic diversity, functional diversity, and related similarity and differentiation measures through hill numbers. Annu. Rev. Ecol. Evol. Syst. 45, 297–324. doi: 10.1146/annurev-ecolsys-120213-091540
Chao, A., and Shen, T.-J. (2003). Nonparametric estimation of Shannon's index of diversity when there are unseen species in sample. Environ. Ecol. Stat. 10, 429–443. doi: 10.1023/A:1026096204727
Condit, R., Pitman, N., Leigh, J., Chave, J., Terborgh, J., Foster, R. B., et al. (2002). Beta-Diversity in Tropical Forest Trees. Science 295:e1066854. doi: 10.1126/science.1066854
De Sousa, A. G.. (1968). Reservas Florestais de Moçambique. Instituto de Investi- gação Agronómica de Moçambique. Comunicação N. ° 10. Apartado 431. Lourenço Marques.
Delgado, J. D., Arroyo, N. L., Arévalo, J. R., and Fernández-Palacios, J. M. (2007). Edge effects of roads on temperature, light, canopy cover, and canopy height in laurel and pine forests (Tenerife, Canary Islands). Landsc. Urban Plan. 81, 328–340. doi: 10.1016/j.landurbplan.2007.01.005
Devlaeminck, R., Bossuyt, B., and Hermy, M. (2005). Inflow of seeds through the forest edge: evidence from seed bank and vegetation patterns. Plant Ecol. 176, 1–17. doi: 10.1007/s11258-004-0008-2
Dobson, A. P., Borner, M., and Sinclair, A. R. E. (2010). Road will ruin Serengeti. Nature 467, 272–273. doi: 10.1038/467272a
Dutton, T., and Dutton, E. (1973). Reconhecimento Preliminar das Montanhas de Chimanimani e Zonas Adjacentes a Criação duma Área de Conservação. Loureço Marques: Repartição Técnica da Fauna de Moçambique, Direcção dos Serviços de Veterinária.
Eriksson, L.. (2020). Assessment of land use change in Moribane Forest Reserve, Mozambique by satellite image classification. MSc thesis, Department of Biological and Environmental Science, University of Jyväskylä. Finland.
Ervin, G. N.. (2009). Portion of its Present US Range Boundary. Southeastern Nat. 8, 293–304. doi: 10.1656/058.008.0208
Fernandes, G. W., Banhos, A., Barbosa, N. P. U., Barbosa, M., Bergallo, H. G., Loureiro, C. G., et al. (2018). Restoring Brazil's road margins could help the country offset its CO2 emissions and comply with the Bonn and Paris Agreements. Perspect. Ecol. Conserv. 16, 105–112. doi: 10.1016/j.pecon.2018.02.001
Follak, S., Eberius, M., Essl, F., Fürdös, A., Sedlacek, N., and Trognitz, F. (2018). Invasive alien plants along roadsides in Europe. EPPO Bull. 2018, 1–10. doi: 10.1111/epp.12465
Fyumagwa, R., Gereta, E., Hassan, S., Kideghesho, J. R., Kohi, E. M., Keyyu, J., et al. (2013). Roads as a threat to the serengeti ecosystem. Conserv. Biol. 2013, 1–4. doi: 10.1111/cobi.12116
Gálhidy, L., Mihók, B., Hagyó, A., Rajkai, K., and Standovár, T. (2006). Effects of gap size and associated changes in light and soil moisture on the understorey vegetation of a Hungarian beech forest. Plant Ecol. 183, 133–145. doi: 10.1007/s11258-005-9012-4
Gaujour, E., Amiaud, B., Mignolet, C., and Plantureux, S. (2012). Factors and processes affecting plant biodiversity in permanent grasslands. A review. Agronom. Sustain. Dev. 32, 133160. doi: 10.1007/s13593-011-0015-3
Gianuca, A. T., Declerck, S. A. J., Lemmens, P., and De Meester, L. (2017). Effects of dispersal and environmental heterogeneity on the replacement and nestedness components of β-diversity. Ecology 98, 525–533. doi: 10.1002/ecy.1666
Givá, N.. (2016). Parks With People? Action Research in Bridging Conservation and Livelihoods. Doutoral Thesis. Swedish University of Agricultural Sciences. Uppsala.
Gogoi, A., Sahoo, U. K., and Saikia, H. (2020). Vegetation and ecosystem carbon recovery following shifting cultivation in Mizoram-Manipur-Kachin rainforest eco-region, Southern Asia. Ecol. Proc. 9:21. doi: 10.1186/s13717-020-00225-w
Goldblum, D.. (1997). The effects of treefall gaps on understory vegetation in New York State. J. Veg. Sci. 8, 125–132. doi: 10.2307/3237250
Gotelli, N. J., and Chao, A. (2013). Measuring and Estimating Species Richness, Species Diversity, and Biotic Similarity From Sampling Data. Encyclopedia of Biodiversity: Second Edition (Vol. 5). Elsevier Ltd. doi: 10.1016/B978-0-12-384719-5.00424-X
Hansen, M. J., and Clevenger, A. P. (2005). The influence of disturbance and habitat on the presence of non-native plant species along transport corridors. Biol. Conserv. 125, 249–259. doi: 10.1016/j.biocon.2005.03.024
Hejda, M., Pyšek, P., and Jarošík, V. (2009). Impact of invasive plants on the species richness, diversity and composition of invaded communities. J. Ecol. 97, 393–403. doi: 10.1111/j.1365-2745.2009.01480.x
Helldin, J. O.. (2019). Predicted impacts of transport infrastructure and traffic on bird conservation in Swedish Special Protection Areas. Nat. Conserv. 36, 1–16. doi: 10.3897/natureconservation.36.31826
Hill, M. J., Heino, J., Thornhill, I., Ryves, D. B., and Wood, P. J. (2017). Effects of dispersal mode on the environmental and spatial correlates of nestedness and species turnover in pond communities. Oikos 126, 1575–1585. doi: 10.1111/oik.04266
Hill, M. O.. (1973). Diversity and evenness: a unifying notation and its consequences. Ecology 54, 427–432. doi: 10.2307/1934352
Hopcraft, J. G. C., Mduma, S. A. R., Borner, M., Bigurube, G., Kijazi, A., Haydon, D. T., et al. (2015). Conservation and economic benefits of a road around the Serengeti. Conserv. Biol. 2015, 1–5. doi: 10.1111/cobi.12470
Hsieh, T. C., Ma, K. H., and Chao, A. (2016). iNEXT: An R package for interpolation and extrapolation of species diversity (Hill numbers). Methods Ecol. Evol. 2016, 1–33. doi: 10.1111/2041-210X.12613
Jones, K. R., Venter, O., Fuller, R. A., Allan, J. R., Maxwell, S. L., Negret, P. J., et al. (2018). One-third of global protected land is under intense human pressure. Science 360, 788–791. doi: 10.1126/science.aap9565
Jost, L.. (2007). Partitioning diversity into independent alpha beta concepts. Ecology 88, 2427–2439. doi: 10.1890/06-1736.1
Kindt, R.. (2019). Package for Community Ecology and Suitability Analysis. Cran. Available online at: https://cran.r-project.org/web/packages/BiodiversityR/index.html
Laurance, W. F., and Balmford, A. (2013). Land use: A global map for road building. Nature 495, 308–309. doi: 10.1038/495308a
Laurance, W. F., Campbell, M. J., Alamgir, M., and Mahmoud, M. I. (2017). Road expansion and the fate of Africa's tropical forests. Front. Ecol. Evol. 5, 1–7. doi: 10.3389/fevo.2017.00075
Laurance, W. F., Goosem, M., and Laurance, S. G. W. (2009). Impacts of roads and linear clearings on tropical forests. Trends Ecol. Evol. 24, 659–669. doi: 10.1016/j.tree.2009.06.009
Lázaro-Lobo, A., and Ervin, G. N. (2019). A global examination on the differential impacts of roadsides on native vs. exotic and weedy plant species. Glob. Ecol. Conserv. 17, 1–13. doi: 10.1016/j.gecco.2019.e00555
Legendre, P.. (2014). Interpreting the replacement and richness difference components of beta diversity. Glob. Ecol. Biogeograp. 23, 1324–1334. doi: 10.1111/geb.12207
Leprieur, F., Tedesco, P. A., Hugueny, B., Beauchard, O., Dürr, H. H., Brosse, S., et al. (2011). Partitioning global patterns of freshwater fish beta diversity reveals contrasting signatures of past climate changes. Ecol. Lett. 14, 325–334. doi: 10.1111/j.1461-0248.2011.01589.x
Lisboa, S. N., Guedes, B. S., Ribeiro, N., and Sitoe, A. (2018). Biomass allometric equation and expansion factor for a mountain moist evergreen forest in Mozambique. Carbon Balance Manag. 13:111. doi: 10.1186/s13021-018-0111-7
Lugo, A. E., and Gucinski, H. (2000). Function, effects, and management of forest roads. For. Ecol. Manage. 133, 249–262. doi: 10.1016/S0378-1127(99)00237-6
Macauhub (2010). Road Between Chimio and Espungabera in Mozambique to be Paved. Available online at: https://macauhub.com.mo/2010/05/28/9162/ (accessed August 2020).
Monteiro, J., Dondeyne, S., Wursten, B., Bannerman, J., Meilby, H., and Sitoe, A. (2011). Vegetation composition of natural gaps in Moribane forest (Mozambique). Afr. J. Ecol. 49, 510–514. doi: 10.1111/j.1365-2028.2011.01281.x
Moreno, C. E., Barragán, F., Pineda, E., and Pavón, N. P. (2011). Reanálisis de la diversidad alfa: Alternativas para interpretar y comparar información sobre comunidades ecológicas. Rev. Mex. Biodivers. 82, 1249–1261. doi: 10.22201/ib.20078706e.2011.4.745
Müller, T., Sitoe, A., and Mabunda, R. (2005). Assessment of the Forest Reserve Network in Mozambique. Maputo: WWF.
Naaf, T., and Wulf, M. (2007). Effects of gap size, light and herbivory on the herb layer vegetation in European beech forest gaps. For. Ecol. Manage. 244, 141–149. doi: 10.1016/j.foreco.2007.04.020
Ngarakana, E., and Kativu, S. (2017). Soil based assessment of the invasive species Vernonanthura phosphorica (Vell.) H. Rob. (Asteraceae) in Burma Valley, Zimbabwe. Transact. R. Soc. South Africa. 73, 16–19. doi: 10.1080/0035919X.2017.1369470
Oksanen, J., Blanchet, F. G., Friendly, M., Kindt, R., Legendre, P., Mcglinn, D., et al. (2019). Community Ecology Package. Package. Vegan 2, 1–297.
Parendes, L. A., and Jones, J. A. (2000). Role of Light Availability and Dispersal in Exotic Plant Invasion along Roads and Streams in the H. J. Andrews Experimental Forest, Oregon. Conserv. Biol. 14, 64–75. doi: 10.1046/j.1523-1739.2000.99089.x
Pauchard, A., and Alaback, P. B. (2004). Influence of elevation, land use, and landscape context on patterns of alien plant invasions along roadsides in protected areas of south-central Chile. Conserv. Biol. 18, 238–248. doi: 10.1111/j.1523-1739.2004.00300.x
Peterson, G., Allen, C. R., and Holling, C. S. (1998). Ecological Resilience, Biodiversity, and Scale. Springer. doi: 10.1007/s100219900002
Pooley, E., and Carruthers, V. (1999). Flowers, Grasses, Ferns and Fungi. Southern African Green Guide. South Africa, Southern Book.
Poorter, L., Bongers, F., and Rozendaal, D. M. A. (2016). Biomass resilience of Neotropical secondary forests. Nature 530, 211–214. doi: 10.1038/nature16512
R Core Team (2018). R: A Language and Environment for Statistical Computing. Vienna: R Foundation for Statistical Computing.
Rendón-Sandoval, F. J., Casas, A., Moreno-Calles, A. I., Torres-García, I., and García-Frapolli, E. (2020). Traditional agroforestry systems and conservation of native plant diversity of seasonally dry tropical forests. Sustainability 12, 1–27. doi: 10.3390/su12114600
Root-Bernstein, M., and Svenning, J. C. (2018). Human paths have positive impacts on plant richness and diversity: A meta-analysis. Ecol. Evol. 8, 11111–11121. doi: 10.1002/ece3.4578
Ruiz-Jaen M. C. Aide T. M. (2005) Restoration success: how is it being measured? Restor. Ecol. 13, 569577. 10.1111/j.1526-100X.2005.00072.x
Seabra, L. R.. (1961). Solos, Clima e Vegetação do Bloco de Sussundenga. Relatório Final do curso de Engenheiro Silvicultor. Universidade Técnica de Lisboa.
Slingenberg, A., Braat, L., Windt, H. Van Der, Rademaekers, K., Eichler, L., and Turner, K. (2009). Study on understanding the causes of biodiversity loss and the policy assessment framework. Final Report. Available online at: https://ec.europa.eu/environment/enveco/biodiversity/pdf/causes_biodiv_loss.pdf
Spehn, E. M., and Korner, C. (2010). Data Mining for Global Trends in Mountain Biodiversity. CRC Press and Taylor & Francis Group, LLC. doi: 10.1201/9781420083705
Spehn, E. M., Liberman, M., and Korner, C. (2006). “Land use change and mountain biodiversity,” in Mountain Research and Development, eds E. M. Spehn, M. Liberman, and C. Korner (New Work, NY: CRC Press and Taylor & Francis Group, LLC). doi: 10.1201/9781420002874
Spooner, P. G., and Smallbone, L. (2009). Effects of road age on the structure of roadside vegetation in south-eastern Australia. Agri. Ecosyst. Environ. 129, 57–64. doi: 10.1016/j.agee.2008.07.008
Suárez-Esteban, A., Fahrig, L., Delibes, M., and Fedriani, J. M. (2016). Can anthropogenic linear gaps increase plant abundance and diversity? Landsc. Ecol. 31, 721–729. doi: 10.1007/s10980-015-0329-7
Tilman, D., Fargione, J., Wolff, B., D'Antonio, C., Dobson, A., Howarth, R., et al. (2001). Forecasting agriculturally driven global environmental change. Science 292, 281284. doi: 10.1126/science.1057544
Timberlake, J.. (2017). Biodiversity Knowledge From the Chimanimani Trans-Frontier Conservation Area (TFCA). Available online at: https://biofund.org.mz/wp-content/uploads/2018/12/1544518689-Biodiversity%20Knowledge%20on%20Chimanimani%20TFCA_FINAL,%20July2017.pdf (accessed 20 September, 2021).
Ullmann, I., Bannister, P., and Wilson, J. B. (1995). The vegetation of roadside verges with respect to environmental gradients in southern New Zealand. J. Veg. Sci. 6, 131–142. doi: 10.2307/3236264
Vajari, K. A., Jalilvand, H., Pourmajidi, M. R., and Espahbodi, K. (2011). The effect of canopy gaps on biodiversity of herbaceous species in an oriental beech (fagus orienatlis lipsky) stand. Res. J. Forest. 5, 36–44. doi: 10.3923/rjf.2011.36.44
van Oudtshoorn, F.. (2012). Guide to Grasses of southern Africa. 3rd edition. Pretoria: Briza Publications.
van Vliet, N., Mertz, O., and Heinimann, A. (2012). Trends, drivers and impacts of changes in swidden cultivation in tropical forest-agriculture frontiers: a global assessment. Global Environ. Change 22, 418–429. doi: 10.1016/j.gloenvcha.2011.10.009
van Wyk, B., and van Wyk, P. (1997). Field Guide to Trees of Southern Africa, 1st edn. Cape Town: Struik Nature.
Venables, W. N., and Ripley, B. D. (2002). Modern Applied Statistics with S, 4th Edn. doi: 10.1214/aoms/1177697510
Votsi, N. E., Mazaris, A. D., Kallimanis, A. S., Zomeni, M. S., Vogiatzakis, I. N., Sgardelis, S. P., et al. (2012). Road effects on habitat richness of the Greek Natura 2000 network. Nature Conservation 1, 53–71. doi: 10.3897/natureconservation.1.2086
Keywords: alpha-diversity, beta-diversity, conservation area, transport infrastructure, biodiversity conservation, environmental impact assessment
Citation: Lisboa SN, Domingos F, Vallius E, Lensu A, Macamo E and Sitoe A (2022) Assessing the Impact of Road and Land Use on Species Diversity of Trees, Shrubs, Herbs and Grasses in the Mountain Landscape in Southern Africa. Front. Conserv. Sci. 3:829690. doi: 10.3389/fcosc.2022.829690
Received: 06 December 2021; Accepted: 14 February 2022;
Published: 31 March 2022.
Edited by:
Jiajia Liu, Fudan University, ChinaReviewed by:
Anatoliy A. Khapugin, Tyumen State University, RussiaTiantian Zhang, Fudan University, China
Copyright © 2022 Lisboa, Domingos, Vallius, Lensu, Macamo and Sitoe. This is an open-access article distributed under the terms of the Creative Commons Attribution License (CC BY). The use, distribution or reproduction in other forums is permitted, provided the original author(s) and the copyright owner(s) are credited and that the original publication in this journal is cited, in accordance with accepted academic practice. No use, distribution or reproduction is permitted which does not comply with these terms.
*Correspondence: Sá Nogueira Lisboa, sanogueiralisboa@gmail.com