Remediation of heavy metals by native plant species grown in Iran’s richest gold mine and study of plants’ pollution tolerance strategies
- 1Department of Ecology and Aquatic Stocks Management, Artemia and Aquacultur Research Institute, Urmia University, Urmia, Iran
- 2Department of Biology, Faculty of Science, Urmia University, Urmia, Iran
- 3Department of Geology, Faculty of Science, Urmia University, Urmia, Iran
Mining is defined as an environmentally hazardous activity that releases metals and other elements to the environment. Bioremediation is a natural and sustainable technique for harnessing the potential of microorganisms and plants to remove, degrade, or stabilize pollutants from contaminated sites and enable cleanup and restoration of the environment. In the present study, following the investigation of pollution of heavy metals in soil samples collected from the Zarshuran mining area, the role of twenty native plant species in the bioaccumulation of heavy metals was evaluated. After preparation of soil and plant samples, inductively coupled plasma-mass spectroscopy (ICP-MS) was used to determine the concentrations of elements in the soil and plant samples. It was confirmed that the soil samples were highly contaminated by silver (Ag), zinc (Zn), cadmium (Cd), lead (Pb), and antimony (Sb). High amounts of Pb and Zn were accumulated in Eryngium billardieri and Astragalus rostratus. Further, these two plant species could uptake, transport, and accumulate Ag in their aerial parts and the enrichment coefficient of their shoots was more than 1. Scorzonera latifolia also had good potential to stabilise Ag, Zn, and Pb in its root. As a result, E. billardieri, A. rostratus, and S. latifolia may be suitable candidate species to clean heavy metals from soils in contaminated sites. Overall, augmentation of superoxide dismutase activity and the amounts of total phenols and flavonoids in different parts of E. billardieri and A. rostratus confirmed the induction of antioxidant defense systems in the plants (compared to the control plants) and an attempt by the plants to tolerate heavy metal pollution.
1 Introduction
Heavy metals are one of the main environmental contaminants with their high accordance as a contaminant, non-biodegradable features, and low solubility in biota, thus posing a high risk to human health (Sheoran, et al., 2016; Sodhi, et al., 2022). They have received considerable attention over the last decades (Shahid, et al., 2017). In addition, the amount of metals in the environment has increased due to mining activities and widespread usage of pesticides and fertilizers (Worlanyo and Jiangfeng, 2021; Yan, et al., 2023). The current physicochemical remediation techniques, such as soil replacement, thermal desorption, electric remediation, and soil leaching, have drawbacks such as energy-extensive consumption and the problem of not being affordable and eco-friendly (Li, et al., 2022).
Phytoremediation can provide an eco-friendly, cost-effective, and long-lasting solution to environmental remediation (Mishra, et al., 2020; Bhat, et al., 2022). Various plant species with different capabilities in the uptake, accumulation, and extraction of heavy metals are widespread throughout the world (Shtangeeva, 2021). Information about plant-soil relationships, the concentration of metals in plants, and their remediation potential is growing continuously (Azizi, et al., 2023).
Bio-monitoring and evaluation of heavy metals in plants can serve as an essential tool to determine plants’ sensitivity and their treatment potential (Zhou, et al., 2008; Cuevas, et al., 2023). Moreover, the assessment of some physiological and biochemical responses of the plants have revealed the pollution levels and toxicity of contaminants (Mansoor, et al., 2023). One of the consequences of heavy metals’ pollution is the induced production of reactive oxygen species (ROS) that can lead to many adverse effects in the plants, such as growth inhibition, hormonal and nutrient imbalances, vacuole shrinkage, chromosomal aberrations, DNA damage (Yan and Chen, 2019; Rajput, et al., 2021), disruption of photosynthetic electron transfer chain, and inhibition of photosystem II (PSII) (Sachdev, et al., 2021), which subsequently leads to alteration of oppression and fluidity of the thylakoids (Espinosa-Vellarino, et al., 2020). In response, plants stimulate antioxidant enzyme activities or increase the content of some non-enzymatic antioxidants to reduce the damage associated with the presence of such pollutants and ROS production. Thus, the investigation of antioxidant responses in plants can be helpful to understand their adaptation mechanisms (Rajput, et al., 2021).
Mining activities in the Zarshuran gold deposit date back hundreds of years and, therefore, a large volume of pollutants has been accumulated in the area (Modabberi and Moore, 2004; Torbati, et al., 2018). There is, however, little information currently on the adverse effects of mining activities in the area and the concentration of elements in native plants which grow there. There has been a growing interest in screening and planting local heavy-metal-tolerant plants and identifying their adaptation mechanisms to pollution, especially in the mining areas of the world (Lu, et al., 2017). For example, the potential of Suaeda glauca, Atriplex canescens, and Artemisia desertorum in phytoextraction and phytostabilisation of copper and zinc was confirmed in Jinchang mining area, China. These plants tended to increase exocrine substances and certain secondary metabolites or enhance the activity of antioxidizing enzymes to defend against stress caused by heavy metals (Li, et al., 2022).
The present study aims to determine the levels of Ag, Cd, Pb, Zn, Cu, and Sb pollution in the soil of the Zarshuran gold mine, to study the performance of 20 plant species in the uptake and accumulation of heavy metals, and to discover the physiological responses (such as changes in activities of superoxide dismutase (SOD) and catalase (CAT) and in the content of phenols and flavonoids) of two indigenous plant species with the best remediation performance. With such information, it may be possible to introduce suitable plant species for cleanup and restoration of contaminated lands resulting from mining activities and to understand the resistance mechanisms of plants against environmental pollution.
2 Geological background of the Zarshuran gold mine
The Zarshuran Carlin-type gold mine, the largest of its kind in Iran and the Middle East’s richest gold deposit, is located 42 km north of Takab city, West-Azarbaidjan province, northwestern Iran (36º 46′ N and 47 08′ E) (Mehrabi, et al., 1999; Tale Fazel, et al., 2023). This mining area is spatially related to the tertiary hydrothermal activities (Aliyari, et al., 2021). According to the reported structural divisions of Iran, the Zarshuran gold mine is a part of the Sanandaj-Sirjan structural zone (Stöcklin, 1968) (Figure 1). The oldest rock units in the Zarshuran mine district are the late Proterozoic-lower Cambrian metamorphic-sedimentary sequence consisting of schist, limestone, dolomite, and shale (Figure 2), called the Iman-Khan complex. Considering bottom-up succession, this complex consists of the Iman-Khan, Chaldagh, and Zarshuran units (Aliyari, et al., 2017). The Iman-Khan unit consists of epidote schist, chlorite schist, and serpentine schist. The Chaldagh unit contains laminated grey-to-cream marble and consists of subhedral to euhedral calcites and dolomites along with framboidal pyrites as open-space fillings. The unit is unconformably overlain by the Iman-Khan unit (Asadi, et al., 2000). The Chaldagh limestone was altered and turned into a porous aggregate in contact with the Zarshuran unit. The Zarshuran unit consists of grey-to-black shale, which was partially metamorphosed to grey-to-black schist. Gold mineralization is mainly associated with Au-bearing pyrite and arsenic-containing pyrite in this unit (Aliyari, et al., 2021). The Cambrian dolostone of the Soltanieh Formation overlies the black shale of the Zarshuran unit. Orebodies generally occur in contact with the Zarshuran black shale and the Chaldagh marble. Furthermore, arsenic mineralization occurs as lenses and veins/veinlets in the Zarshuran unit (Asadi, et al., 2000). The Oligocene porphyritic andesitic rocks are cropped out in the southwestern part of the Zarshuran mining area. The Miocene marl and shale of the Qom Formation and the Quaternary travertines and recent alluvial sediments are the youngest rock units in the mining area.
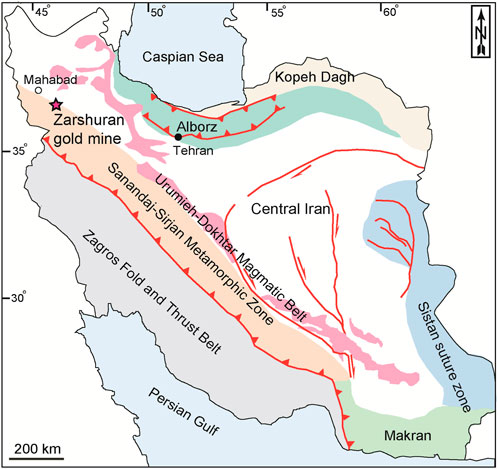
Figure 1. Simplified tectonic map of Iran showing the location of the Zarshuran gold mine, northwestern Iran (Stöcklin, 1968).
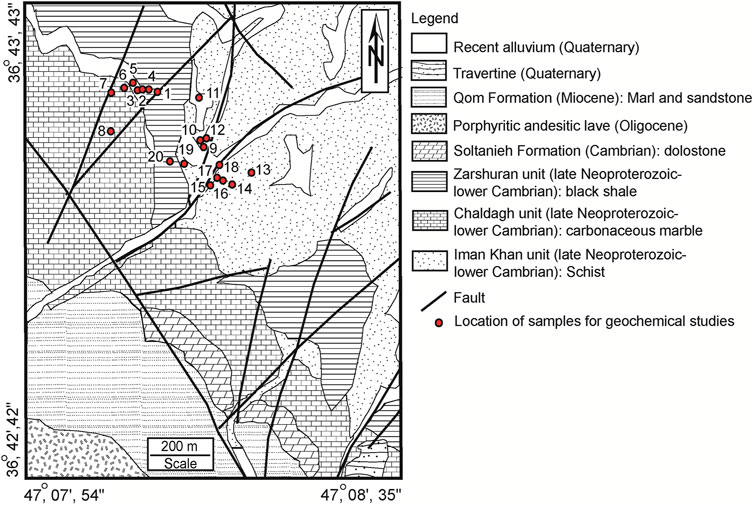
Figure 2. Geological map of the Zarshuran mining district (Tale Fazel, et al., 2023) and the sampling locations in the mining area.
3 Materials and methods
3.1 Metal content in soil and plant samples
The Zarshuran mining area has a semi-arid climate with moderate summers and very cold winters. Its average annual temperature and annual precipitation are about 9°C and 400 mm (falling mostly as snow), respectively (Karimi, et al., 2009). During the spring of 2021, soil and plant samples were collected from the area.
To determine the pollution of six metals (Ag, Cd, Pb, Zn, Sb, and Cu), 20 soil samples were collected from different places in the mining area (36º 43′ N, 47º 08′ E) and from the 20–40 cm depth around the root of the studied plant species. After drying the soil samples at an ambient temperature, they were ground, passed through a 2-mm mesh sieve, and digested using a standard method of digestion (ISO 14869-1) (Shahbazi and Beheshti, 2019). Accordingly, a proper combination of HNO3/HClO4/HF/HCl was used for digestion of soil samples during several repeated steps, and after cooling, the samples were filtered and distilled water was added. They were then stored at 4 °C for further analysis by ICP-MS (Perkin Elmer ELAN 9000) (Torbati, et al., 2018). The pH and EC values of the soil samples were measured by a multi-parameter probe (HI9811-5, Hanna Instrument, Romania) after 20 g of the soil was stirred in 60 mL of distilled water and allowed to stand for about 30 min.
Twenty plant species (each species with three replicates) were then gathered from the studied area according to the national guidelines and legislation. Each time, the samples related to one plant species were taken from the three individual plant species grown in three places close to each other at the same time. The collected plant species were identified by Dr. Esmailbegi Kermani, a plant taxonomist at Urmia University (and one of the authors), and their specimens were deposited with herbarium voucher numbers (Table 1) at the Herbarium of Urmia University, Biology Department. Figure 3 shows the studied plant species in the mining area (Torbati and Esmailbegi Kermani, 2022). Plant samples were thoroughly washed with tap and distilled water. After separating the plants’ roots and their above-ground parts, they were dried at an ambient temperature for 2 weeks. In order to digest and prepare the dried plant samples for ICP-MS, Multiwave 3,000, as a modular microwave system, was used according to the EPA method 3,052 (US EPA Method 3,052, 2019). Accordingly, the plant samples were digested in HNO3-HCl-H2O2 for 15 min in microwave vessels using the microwave system. After cooling, the vessel contents were filtered and then decanted, diluted to a proper volume, and analyzed by ICP-MS.
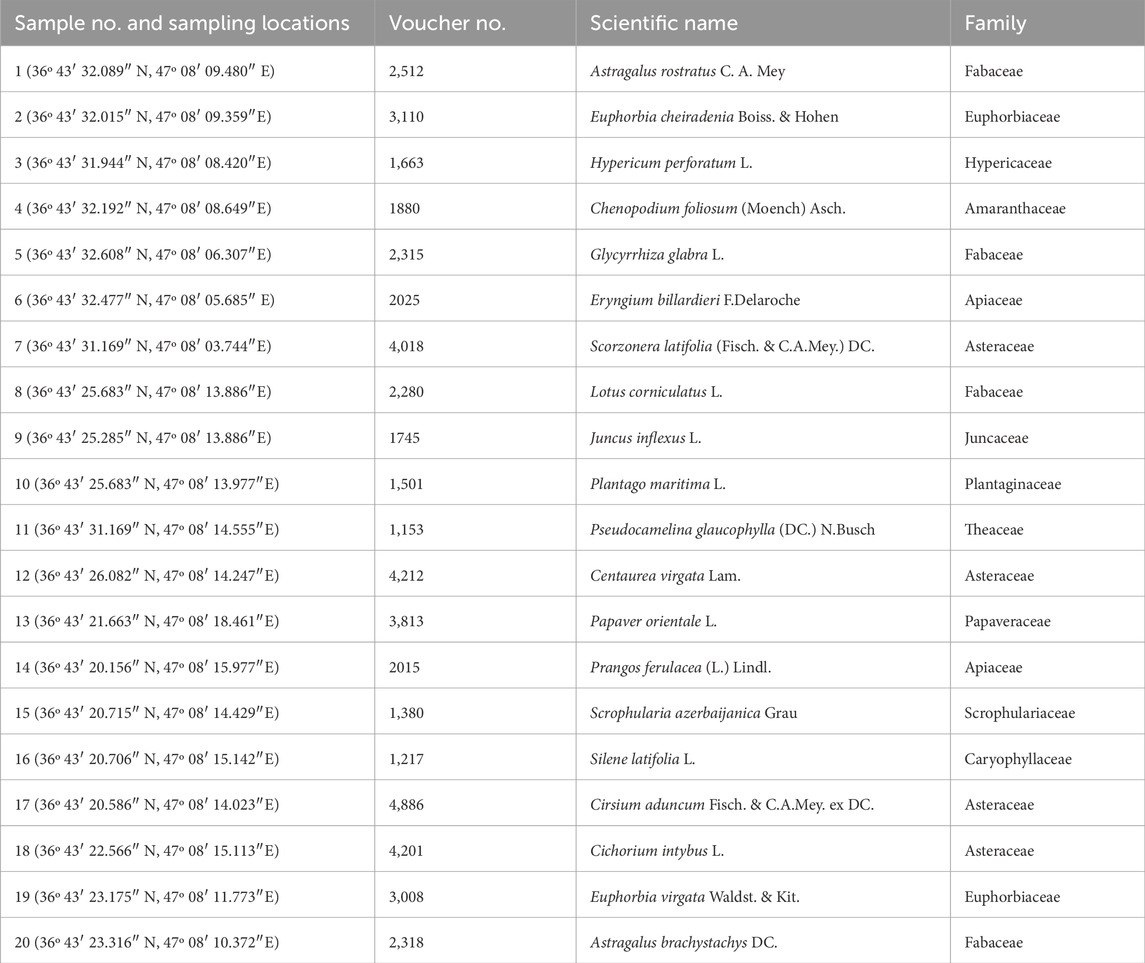
Table 1. Sampling locations, scientific names of collected plant species, their family names, and voucher numbers.
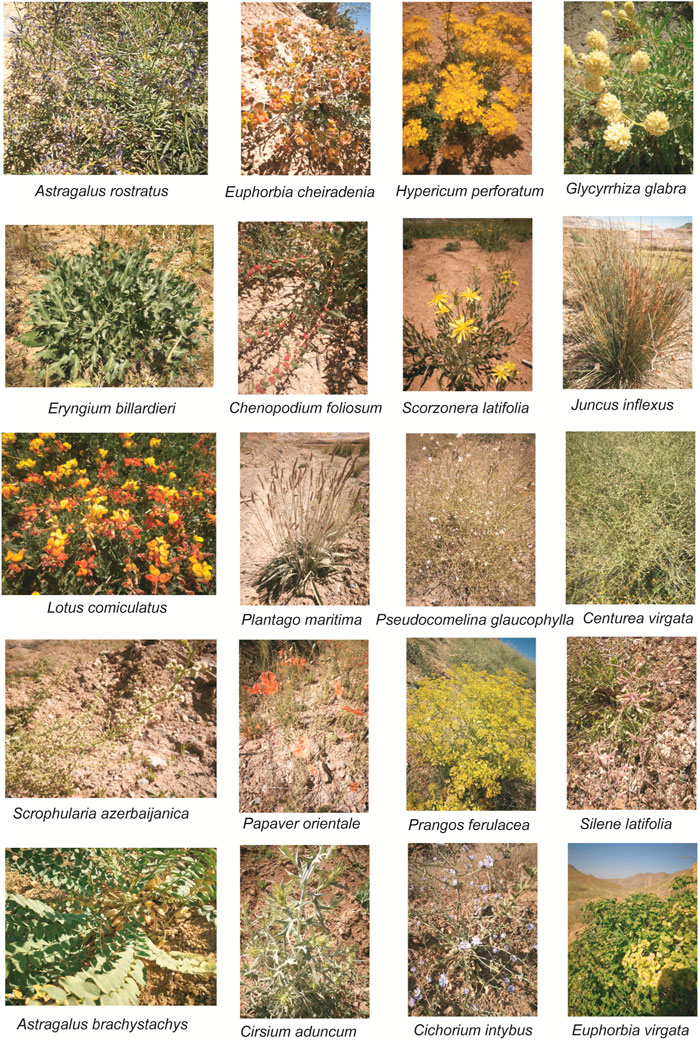
Figure 3. Studied plant species grown in the Zarshuran gold deposit (Torbati and Esmailbegi Kermani, 2022).
After the selection of appropriate plant species according to their general remediation performance and dominant distribution, the control samples were collected about 1–4 km from the mine (36º 42′ N, 47º 06′ E) to compare their biochemical responses with those of the plants grown in the polluted soils. Some plant samples were kept at −85°C for biochemical analyses.
The plant species were identified using reliable botanical sources such as Flora Iranica (Rechinger, 1963) and then compared with other flora of the region, including Flora of Turkey (Davis, 1984) and Flora of Iraq (Townsend et al., 1966).
3.2 Pollution level of the soil
Enrichment factor (EF) as a pollution index was used to evaluate the pollution status of Ag, Cd, Pb, Zn, Cu, and Sb in the soil samples. To calculate EF, the normalization of one metal content to the reference element concentration was needed (Bastami, et al., 2012). Because of the remarkably stable amounts of Iron (Fe) in different soils, it was used as a reference element (RE). Eq. 1 was applied to calculate EF, considering the average abundance of the metals in the Earth’s crust (Taylor, 1964).
3.3 Plants’ bioaccumulation potential
3.3.1 Enrichment coefficients and translocation factor of the plants
The enrichment coefficient of shoot (ECS) is determined by dividing the concentration of the element in the shoot by its concentration in the soil. ECS indicates the plant’s phytoremediation potential. The enrichment coefficient of root (ECR) was expressed as the ratio of the root concentration of the element to that of the element concentration in the soil (Sasmaz, 2008). Plants with high amounts of ECR are suitable for phytostabilisation of elements. The translocation factor (TF) was the ratio of the element in the shoot to the root (Sarwar, et al., 2017).
3.4 Antioxidant activity assays
To perform physiological and biochemical assays, the roots and shoots of two plant species, namely, Eryngium billardieri and A. rostratus, were separately homogenized in the phosphate buffer solution to obtain the crude extracts.
Inhibition of the photochemical reduction rate of nitro blue tetrazolium (NBT) by the plants’ extracts was used for the SOD activity assay (Winterbourn, et al., 1976).
Dismutation of H2O2 at 240 nm was measured to determine the CAT activity (ɛ= 39.4 mM-1 cm-1) (Obinger, et al., 1997).
Total phenols were calculated according to the method reported by Singleton et al. (Singleton, et al., 1999) (Eq. (2)) using the standard gallica acid (GA) graph (R2 = 0.990).
The aluminum chloride colorimetric method was then applied to determine flavonoids content (Chandra, et al., 2014) using the quercetin (QE) calibration curve at 420 nm.
3.5 Statistical analysis
Statistical analyses of the obtained data were performed by one-way analysis of variance (ANOVA) (data with three replicates; using GraphPad Software, Inc. United States of America). The multiple comparisons test was Tukey-Kramer. Significant difference was reported when the probability was less than 0.05 (p < 0.05).
4 Results
4.1 Metal content in the soil samples
Table 2 illustrates the characteristics of 20 soil samples, the elemental concentrations (mg/kg) and enrichment factors (EF) for six elements, and concentration of the elements in control soil samples. Mean electrical conductivity (EC) and pH for the soil samples were 0.27 m/cm and 7.6, respectively. The concentration of the examined elements in soil samples were variable and ranged from 0.1–6.4 mg/kg, 0.3–86.2 mg/kg, 4–2066 mg/kg, 24–7,117 mg/kg, and 14–91 mg/kg for Ag, Cd, Pb, Zn, and Cu, respectively (Table 2). Moreover, Sb content in the soil samples of some locations was more than 100 mg/kg.
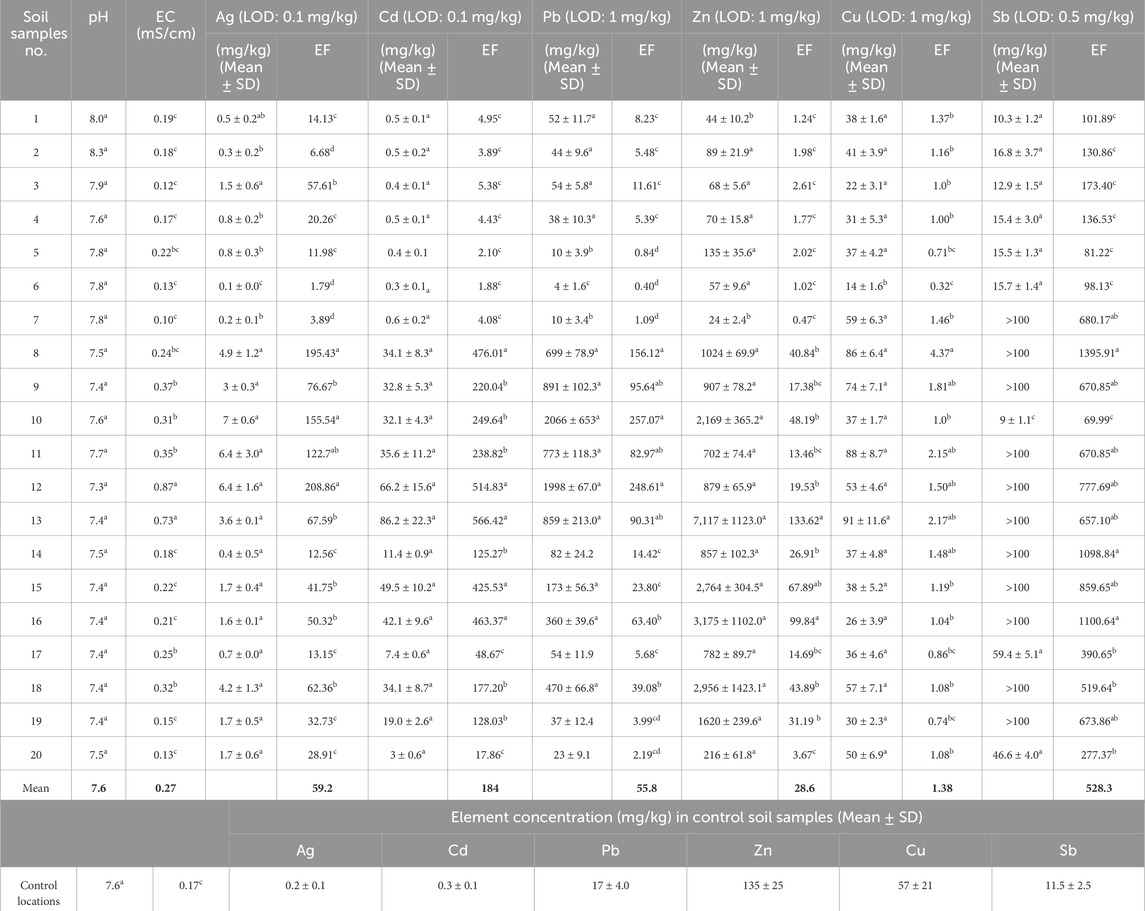
Table 2. EC, pH, concentration of the elements in soil samples (mg/kg), and EF of examined elements [Different letterswithin a columnindicate significant differences(p< 0.05)]. The mean amounts of H, EC and EFs were provided as bold values.
4.2 Metals content of the plants
According to Table 3, Ag content in shoots and roots of E. billardieri (0.8 and 2.4 mg/kg, respectively) and its enrichment coefficients for these parts (8 and 24, respectively) were determined to be the highest compared to the other plants (Table 3). Moreover, ECS and the translocation factor (TF) for A. rostratus and Prangos ferulacea (L.) Lindl. were determined to be more than 1 (1.6 and 4 for A. rostratus and 1 and 4 for P. ferulacea, respectively).
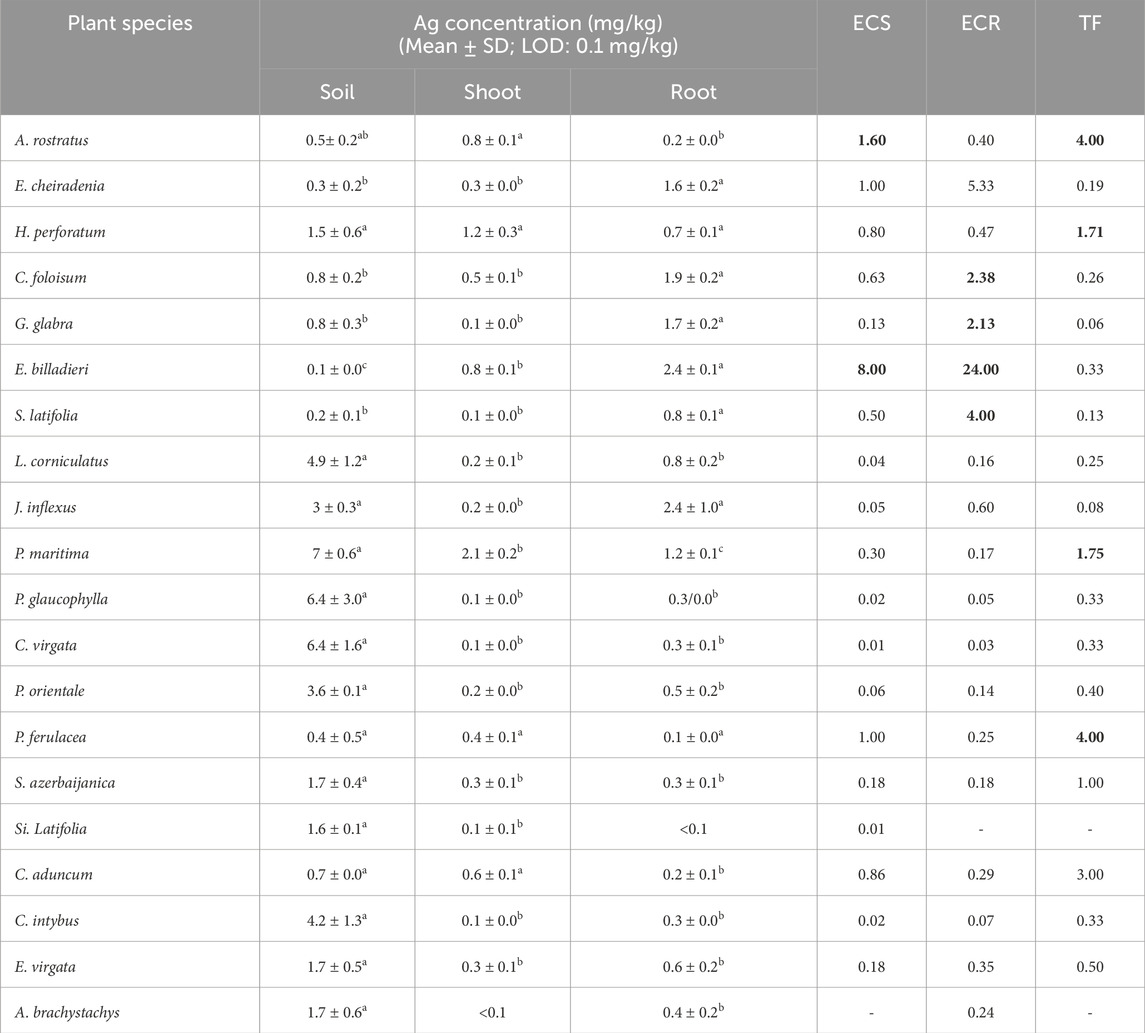
Table 3. Ag concentration (mg/kg) in soil, shoot, and root, enrichment coefficient of shoot (ECS), root (ECR), and translocation factor (TF) of Ag in studied plant species (Different letters within a row indicate significant differences (p < 0.05) (Torbati and Esmailbegi Kermani, 2022). The amounts more than 1 for ECS, ECR and TF were provided as bold values.
The determined ECR for Cd in A. rostratus and Chenopodium foliosum (Moench) Asch. was 1 and the amount of Cd in their roots was equal to that in the soil (Table 4). Although the highest amount of this element in the shoot and root organs among the studied plants was related to Juncus inflexus L. (2 and 11.7 mg/kg, respectively), the performance of the plant in uptake and bioaccumulation of Cd was determined as weak when considering the amount of this element in the related soil sample.
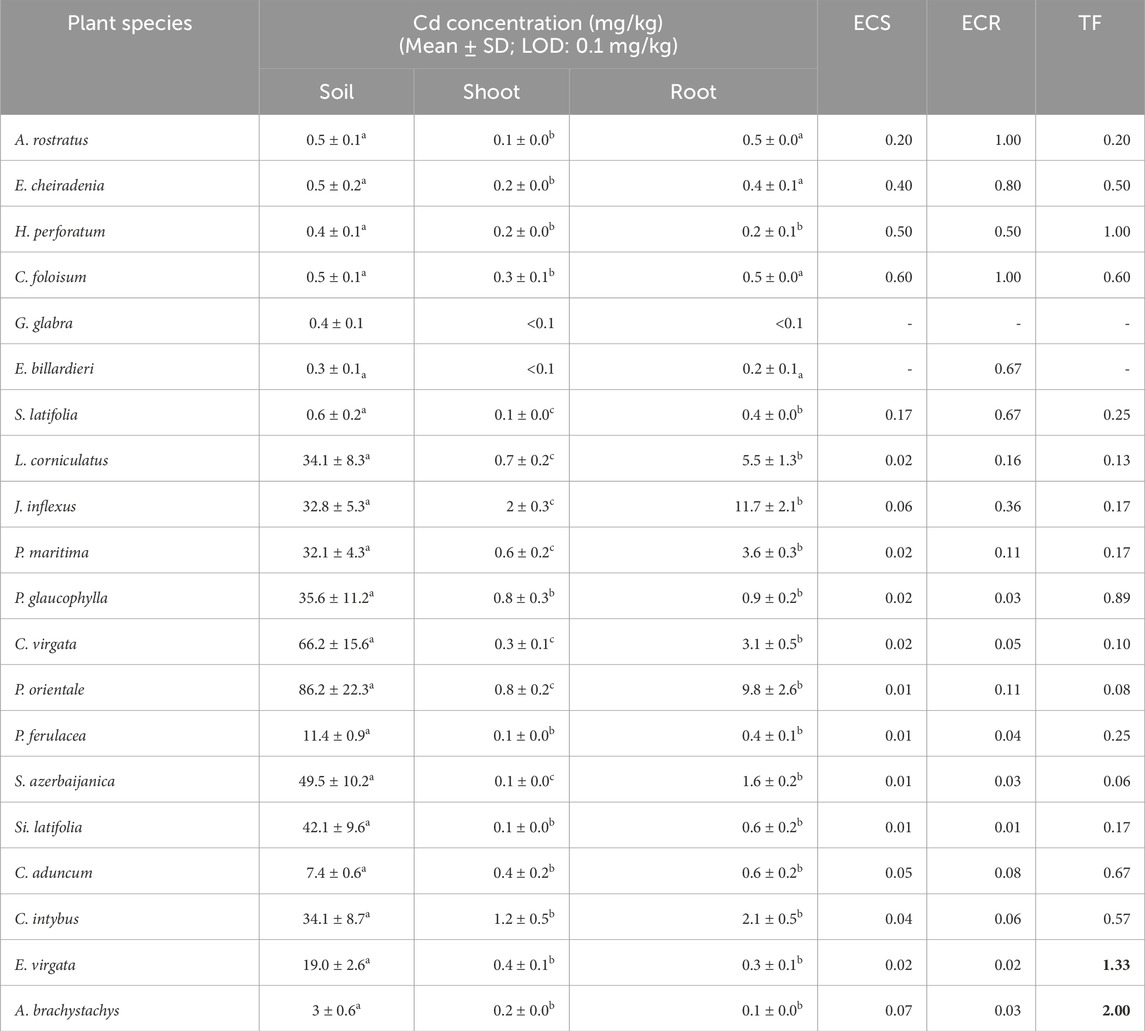
Table 4. Cd concentration (mg/kg) in soil, shoot, and root, enrichment coefficient of shoot (ECS), root (ECR), and translocation factor (TF) of Cd in studied plant species (Different letters within a row indicate significant differences (p < 0.05). The amounts more than 1 for ECS, ECR and TF were provided as bold values.
As shown in Table 5, ECR for Pb in A. rostratus and Euphorbia cheiradenia Boiss. & Hohen. was 1.21 and 1.23, respectively. Furthermore, both ECR and ECS of Pb for Glycyrrhiza glabra L., E. billardieri, Scorzonera latifolia (Fisch. & C.A.Mey.) DC. were more than 1.
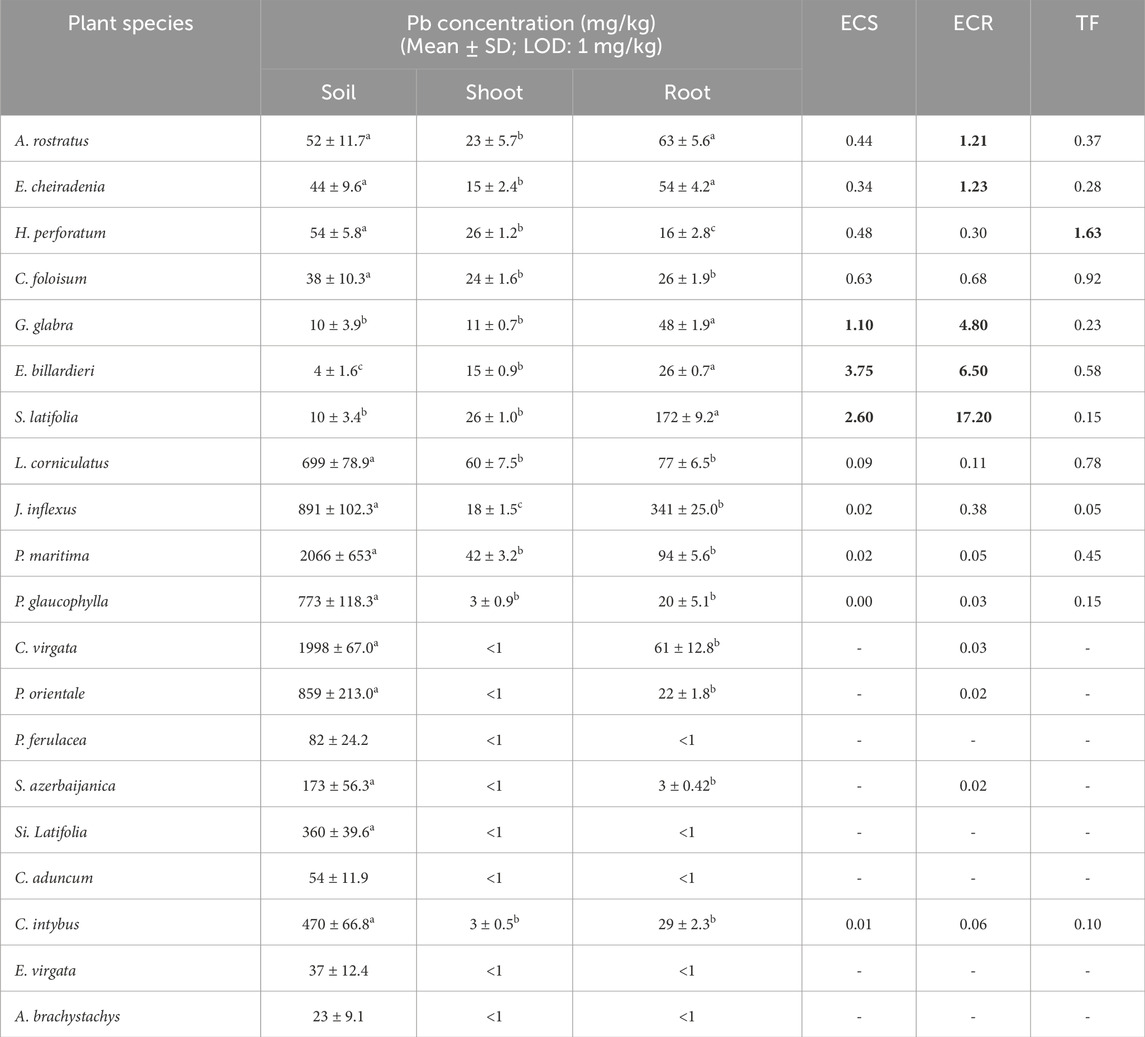
Table 5. Pb concentration (mg/kg) in soil, shoot, and root, enrichment coefficient of shoot (ECS), root (ECR), and translocation factor (TF) of Pb in studied plant species (Different letters within a row indicate significant differences (p < 0.05) (Torbati and Esmailbegi Kermani, 2022). The amounts more than 1 for ECS, ECR and TF were provided as bold values.
Although the studied area was polluted with an average value of 1282.75 mg/kg of Zn, ECR and ECS were more than 1 only in E. billardieri (1.19 and 1.14, respectively) and TF of the plant was approximately 1. The plant had a good performance in terms of the uptake and translocation of Zn to its aerial parts (Torbati and Esmailbegi Kermani, 2022). Moreover, ECR of Zn was 1.59 and 4.67 in A. rostratus and S. latifolia, respectively (Table 6).
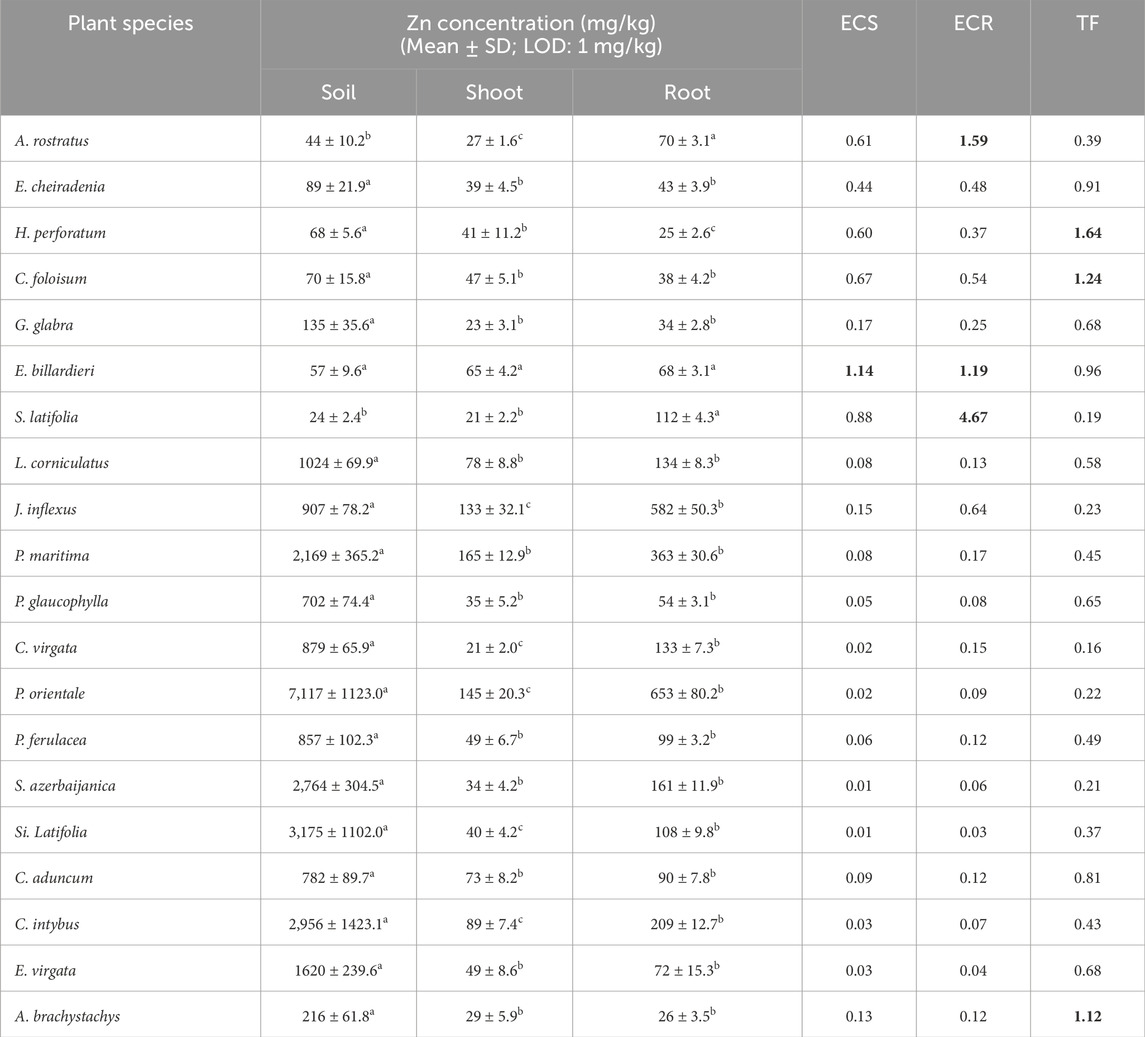
Table 6. Zn concentration (mg/kg) in soil, shoot, and root, enrichment coefficient of shoot (ECS), root (ECR), and translocation factor (TF) of Zn in studied plant species (Different letters within a row indicate significant differences (p < 0.05) (Torbati and Esmailbegi Kermani, 2022). The amounts more than 1 for ECS, ECR and TF were provided as bold values.
Cu amounts of soil samples were variable and its mean amount in the soil samples was 47.25 mg/kg (Table 2). Thus, there was minor enrichment of Cu in soil samples. According to Table 7, ECS and ECR in E. billardieri were 1 and 1.7, respectively. Although the amount of this element in the aerial parts of Centaurea virgata Lam., Papaver orientale L., P. ferulacea, Scrophularia azerbaijanica Grau., Silene latifolia L., and Cirsium aduncum Fisch. & C.A.Mey. ex DC. was higher than in the roots and these plants had a transfer factor of more than 1 (Table 7), none of the mentioned plants could enrich this element in the roots or the aerial parts.
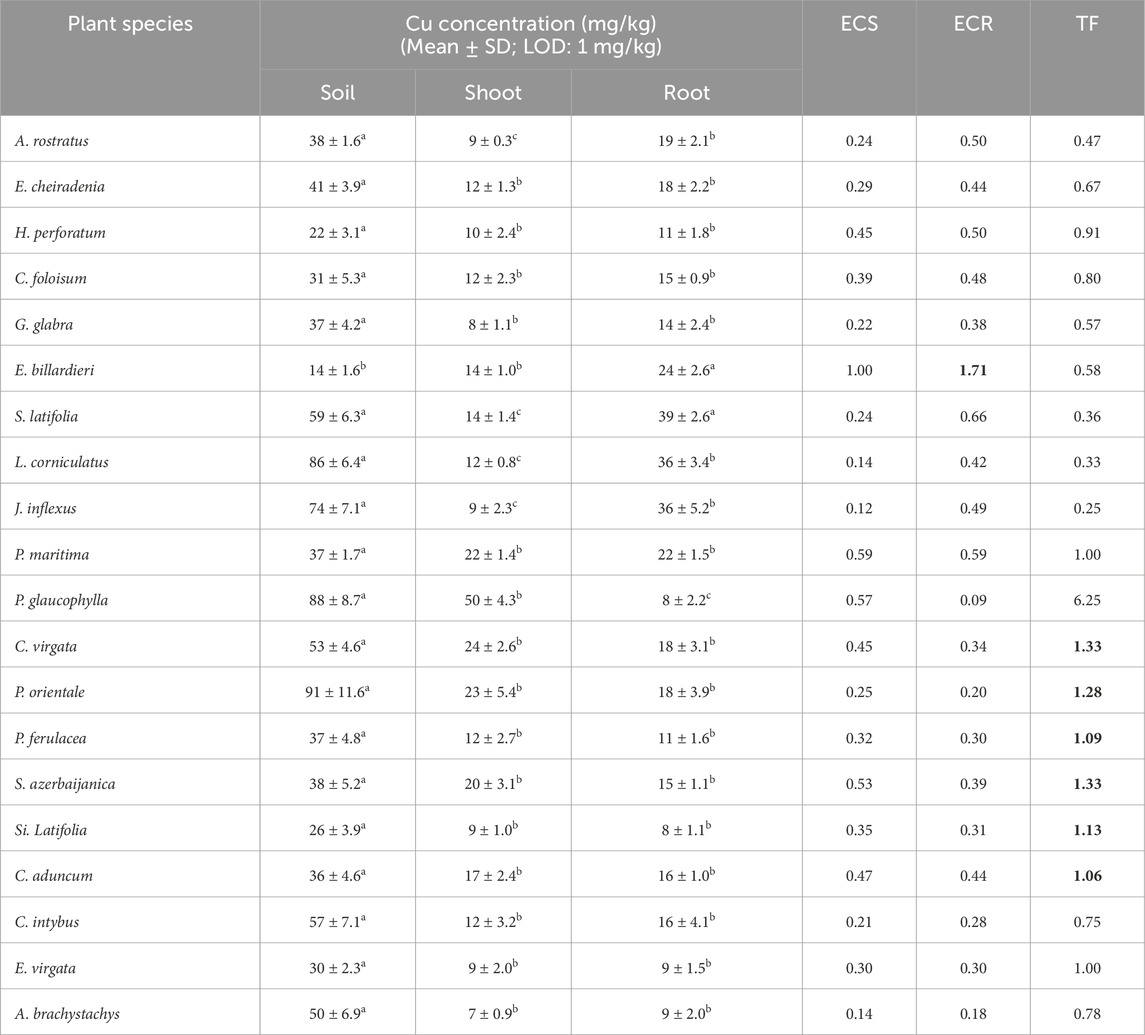
Table 7. Cu concentration (mg/kg) in soil, shoot, and root, enrichment coefficient of shoot (ECS), root (ECR), and translocation factor (TF) of Cu in studied plant species (Different letters within a row indicate significant differences (p < 0.05). The amounts more than 1 for ECS, ECR and TF were provided as bold values.
The mean amount of antimony (Sb) in the soil samples of the Zarshuran mine was 528.26 mg/kg, while the mean concentration in the Earth’s crust is 0.2 mg/kg (Kabata-Pendias, 2010). Despite the high pollution of Sb in the studied area, amounts of ECS and ECR for the examined plant species (Table 8) were more than 1 only in Plantago maritima L. Uptake and transporting abilities of other examined plant species were evaluated as weak. However, root performance in accumulation of Sb was better than in shoot. The amount of Sb in the aerial parts of some studied plant species was determined to be lower than the detection limit of the analysis method (Table 8). Its amount in the roots of the studied plants was determined to be between 1.1 and 75 mg/kg.
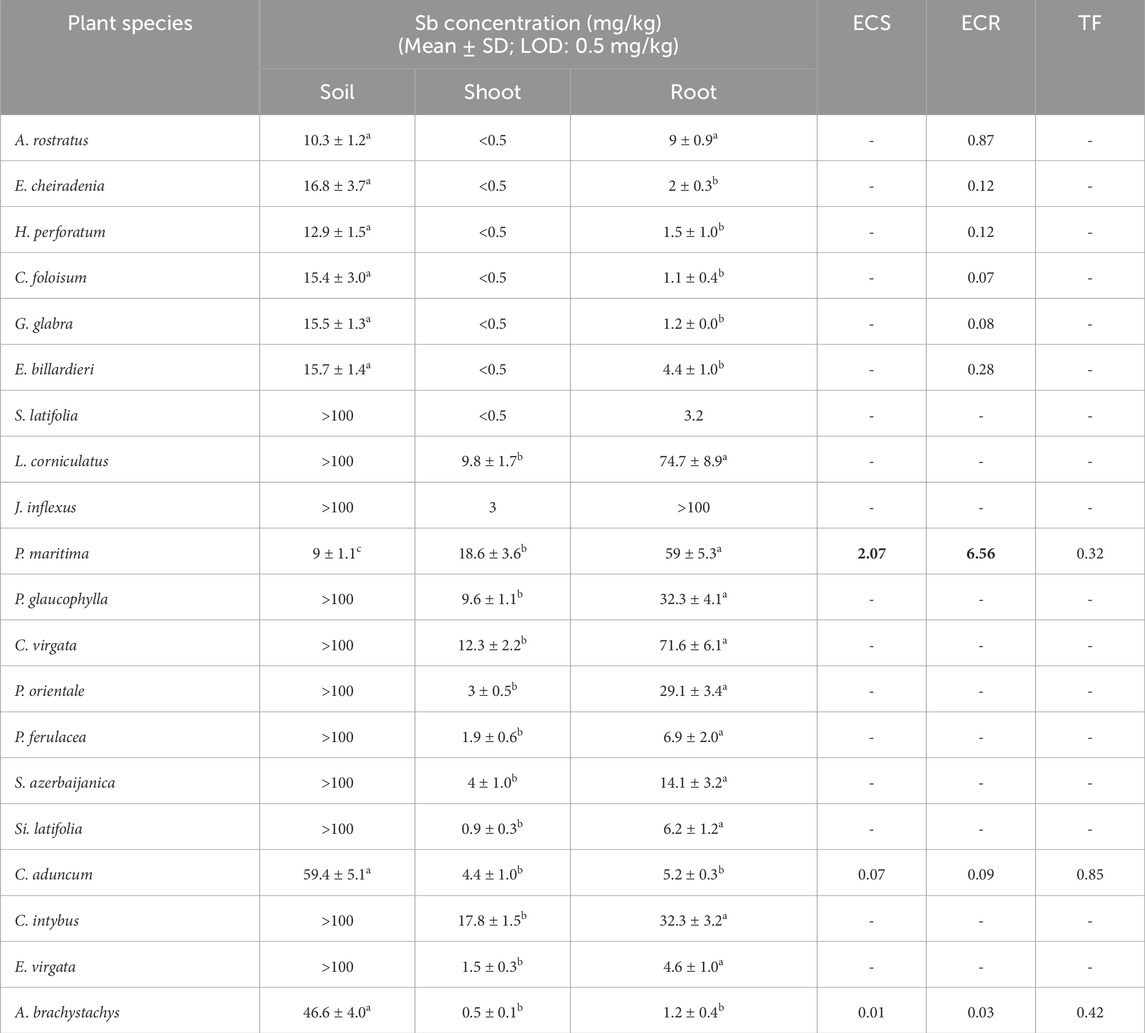
Table 8. Sb concentration (mg/kg) in soil, shoot, and root, enrichment coefficient of shoot (ECS), root (ECR), and translocation factor (TF) of Sb in studied plant species (Different letters within a row indicate significant differences (p < 0.05). The amounts more than 1 for ECS, ECR and TF were provided as bold values.
4.3 Enzymatic and non-enzymatic antioxidant responses
Considering the uptake and accumulation of the examined metals by the plant species and their distribution in the mining area, we selected two plant species, E. billardieri and A. rostratus, for biochemical assays to better understand their tolerance mechanism when confronting the metals’ pollutants in their environment. Their biochemical and physiological responses were compared with those of the control plants grown in the soil with low pollution and about 1–4 km away from the active mining area.
According to Figure 4A, SOD activity in the roots and leaves of E. billardieri was significantly more than that in the control plant (up to 1.5- and 1.4-fold, respectively; p < 0.05). Moreover, the enzyme activity in roots and leaves of A. rostratus was induced near to 1.5-fold more than the control plant (Figure 4B). It seems that the exposure of the plants to pollution from metals in the mining area caused the induction of their SOD activity. The activity of CAT in E. billardieri was similar to the function of SOD (Figure 4A). Its activity was induced notably in the leaves and roots of the plant grown in the mining area as compared to the control plant. Although the increase in CAT activity was also determined for A. rostratus, the enhancement was not significant (p > 0.05) (Figure 4B).
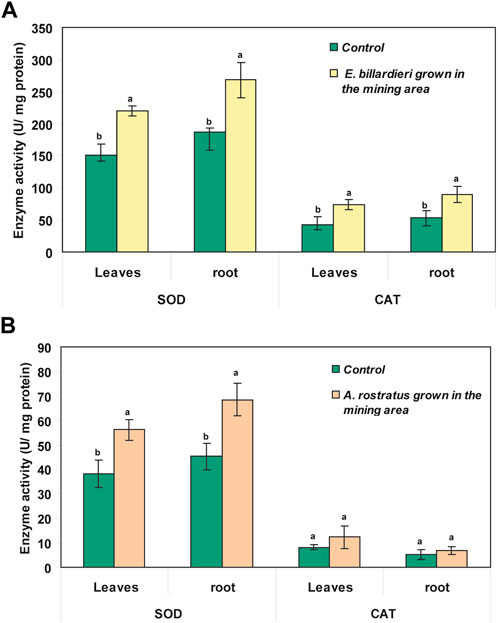
Figure 4. SOD and CAT activities in different parts of (A) Eryngium billardieri and (B) A. rostratus grown in the mining area in comparison with control plants [mean ± SD, n = 3, different letters in each part of one plant species show statistically significant difference (p < 0.05)].
Total phenols and flavonoids in different parts of E. billardieri were about 30% more than those in the control plants (p < 0.05) (Figure 5A). However, the increase of the flavonoid contents in the leaves of E. billardieri was not statistically significant (p > 0.05). The contents of phenols and flavonoids in different parts of A. rostratus grown in the polluted areas were more than those in the control plants (Figure 5B). The increase in total phenol of A. rostratus was about 25% for both leaves and root and flavonoid contents were induced up to 37% and 25% for leaves and root, respectively (as compared to the control plants; Figure 5B). However, the increase in flavonoid content of roots was not significant when compared to the control sample (p > 0.05). In contrast to the high amounts of phenol and flavonoids in the root of E. billardieri, those in the root of A. rostratus were less than the values recorded for its leaves (Figures 5A,B).
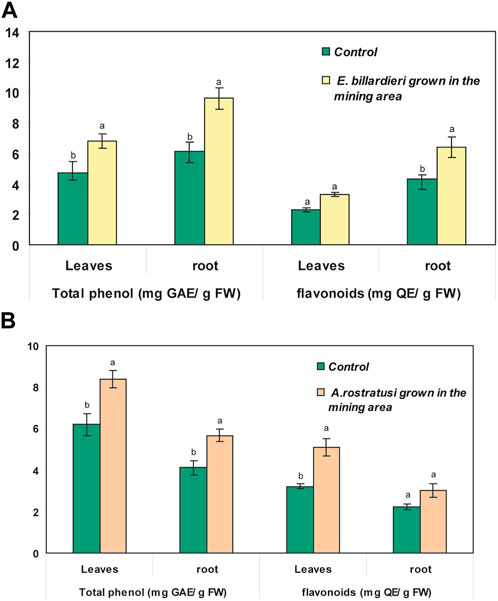
Figure 5. Content of total phenols and flavonoids in different parts of (A) Eryngium billardieri and (B) A. rostratus grown in the mining area in comparison with control plants [mean ± SD, n = 3, different letters in each part of one plant species show statistically significant difference (p < 0.05)].
5 Discussion
5.1 Metal content in the soil and plants
Weathering of minerals and mining activity for many years in the Zarshuran mining area have caused the elevated levels of heavy metals in the soil. According to the obtained results, there was very intense enrichment of Ag, Cd, Pb, Zn, and Sb in the studied area, especially in sampling sites 8-16 (EF for the elements was very high). As described by Muller’s classification (Muller, 1969), EF values more than 10 show severe enrichment (Anbuselvan, et al., 2018). There was also minor enrichment of Cu in the soil samples. In soils highly contaminated by heavy metals, the growth of all but the most tolerant plants is restricted. In addition, several factors like soil texture and pH, redox potential, cation exchange capacity, and plant biomass and root exudates can affect the phytoavailability of metals for plants (Sheoran, et al., 2016).
The time of sample collection, Ag content of the soil, and the plant species are three main factors that can affect the concentration of silver in plants (Kabata-Pendias, 2010). According to our previously presented results, the performance of E. billardieri in the accumulation of significant quantities of Ag in its body was remarkable, especially in its root (Torbati and Esmailbegi Kermani, 2022). The enrichment coefficients of shoot (ECS) and root (ECR) for the plant showed that the plant had a significant potential in phytostabilisation of Ag. A. rostratus also had a good potential in transporting Ag to its aerial parts. According to the previous reports, plants exhibiting TF and ECS values >1 are suitable for phytoextraction (Yoon, et al., 2006). Thus, A. rostratus is a suitable candidate for phytoextraction of Ag. In addition, ECS and TF values of P. ferulacea showed that the plant could be suitable for Ag phytoextraction. Although Brassicaceae has been introduced as a significant Ag accumulator plant family (Sheoran, et al., 2016), detailed information about Ag hyperaccumulator plants is rare. For instance, it has been reported that accumulation of Ag by Atriplex nummularia in its leaves is nearly 46.69 mg/kg more than its concentration in the desert soil (Guda, et al., 2020). The potential of Medicago sativa and Brassica junceae in accumulation of silver from an aqueous substrate containing more than 1,000 mg/kg Ag has already been confirmed (Harris and Bali, 2008).
The average concentration of Cd in the Earth’s crust is 0.1 mg/kg (Ehrampoush, et al., 2015). The obtained average amount of Cd in the study area was 22.8 mg/kg. Despite the high pollution of Cd in the area, only A. rostratus and C. foliosum showed an almost acceptable performance in the uptake and accumulation of Cd to their roots (ECR = 1). In addition to A. rostratus from the Fabaceae family, the potential of some other genera of the family, such as Vigna radiata, in the uptake and accumulation of Cd has been proven in other previous studies (Anjum, et al., 2014). More than A. rostratus, ECR of C. foliosum (Moench) Asch was 1 and the amount of the element in the root was equal to that in the soil. Accumulation of a high amount of Cd in the root of Cd-tolerant plants has also been reported previously (Belimov, et al., 2018). Vacuolar compartmentation of Cd, cytoplasmic chelation, and its adsorption to the cell wall are different strategies used by plants for adaptation to the presence of Cd pollutants (Luo and Zhang, 2021).
Pb has no essential and beneficial role in plants, and its high concentration can create morphological, physiological, and biochemical problems (Collin, et al., 2022). Considering ECR and ECS amounts, G. glabra L., E. billardieri, and S. latifolia concentrated a large amount of Pb in their roots (US EPA, 2019); they could also successfully translocate Pb into their aerial parts. According to the reported categorization of the plants based on the amount of ECR (Egendorf, et al., 2020), the three mentioned plant species along with A. rostratus and E. cheiradenia are phytostabilisers of Pb and could accumulate Pb in their roots. In addition the Pb remediation potential of A. rostratus, the considerable ability of some other plants from the Fabaceae family to uptake and accumulate Pb has also been confirmed previously. For example, the bioaccumulation capability of guar (Cyamopsis tetragonoloba) at higher Pb levels was evaluated as efficient (Amin, et al., 2018). However, according to the literature, only a few plant species were evaluated as plants capable to uptake Pb above the threshold of 1,000 mg/kg (phytoextraction) and none of them could be confirmed as Pb hyperaccumulators (Egendorf, et al., 2020). In contrast, phytostabilisation or accumulation of Pb in the plants’ root is an effective method to remove Pb and to limit Pb mobility in the environment (Collin, et al., 2022). In addition, considering the pH in the sampled soils (7.3−8.3), phytoavailability of Pb was limited because the element is immobile in high pH soil (Honda, et al., 2007).
Zinc is an essential microelement that serves many important roles in plant growth and development. Most plants contain 10–200 mg/kg Zn in their bodies (Balafrej, et al., 2020). The Zn content of plants in this study ranged from 21–653 mg/kg and the maximum value was found in the root of P. orientale. According to Table 6 and amounts of ECR, E. billardieri, A. rostratus, and S. latifolia can be good stabilizers of Zn in their roots. Besides A. rostratus, a high accumulation of Zn in the root of another species of the Fabaceae (Anthyllis vulneraria), up to more than 10,000 mg/kg, has been previously confirmed (Reeves, et al., 2018). However, the related literature describes 28 Zn-hyperaccumulating plant species and most of them belong to the Brassicaceae family (Balafrej, et al., 2020). For instance, Arabidopsis halleri and Noccaea caerulescens were two plant species of Brassicaceae that can accumulate extremely high concentrations of Zn (more than 10,000 mg/kg) when grown in Zn-enriched soils (Peer, et al., 2006).
Copper, an essential metal in plants, plays important roles in plant physiological and biochemical processes, such as photosynthesis and respiration, nitrate metabolism, water permeability, and reproduction. (Kabata-Pendias, 2010). Concentration of Cu in the soil sample in the Zarshuran mining area was approximately in the range of the reported Earth’s crust Cu concentration (25–75 mg/kg) (Kabata-Pendias, 2010). Typically, Cu concentration in plant tissues ranges from 5 to 30 mg kg−1 (Napoli, et al., 2019) and concentration more than 100 mg/kg in plants are rare even in the presence of high soil Cu concentration (Ghaderian and Ghotbi Ravandi, 2012). Among 20 plant species in the present study, only E. billardieri had ECS and ECR ≥1; this means that the plant may have good potential in the uptake and accumulation of Cu, especially in its root (phytostabilisation). However, some plant species such as Aeolanthus biformifolius, Commelina communis, Ipomea alpine, Eleocharis acicularis, Artemisia argyi, Haumaniastrum katangense, and Rumex acetosa have been discovered to be Cu-hyperaccumulators (Napoli, et al., 2019).
There was very intense pollution of Sb in the Zarshuran mining area. Reduction of growth, disruption of uptake of essential mineral nutrients in plants, ROS production, instability of membranes, and induction of other structural changes in plants are some of the adverse effects of Sb toxicity in plants (Tang, et al., 2022). Despite the non-essentiality and toxicity of Sb, some plants can uptake and accumulate Sb in relatively high concentrations in their organs, but a smaller quantity of this metalloid can be translocated to the shoot (Vidya, et al., 2022). In the present study, most Sb was stored in root tissues of the plant species and the amount of Sb in the aerial parts of some studied plant species was undetectable. It was reported that the mean Sb content of terrestrial plants is 0.06 mg/kg, and some plant species such as Barbarea verna, Sorghum bicolor, Lyocopu lucidus, Sambucus chinensis, and Patrinia villos have been previously suggested as potential phytoremediators of Sb (Zhang, et al., 2021; Bolan, et al., 2022).
To summarize, E. billardieri’s potential for the accumulation of Ag, Pb, Zn, and Cu in its roots and shoots was acceptable and the plant could be a good candidate for phytostabilisation of the mentioned elements. ECR of A. rostratus was ≥1 for Cd, Pb, and Zn and the plant could stabilize the elements in its root. Moreover, A. rostratus had ECS and TF of more than 1 for Ag and could extract the element from the soil and transport it to its above-ground parts. S. latifolia had ECR >1 for Ag, Zn, and Pb and the amount of ECS of the plant for Pb was also more than 1.
Although phytoremediation is an effective and eco-friendly remediation technology, the disposal of a large number of hyperaccumulator plant harvests has become a significant problem that should be solved. Solid waste treatment methods, such as composting processes, ashing, incineration, and liquid phase extraction, are some traditional disposal techniques (Fu, et al., 2021). In contrast, synthesis of nanoparticles, hydrothermal upgrading, and phytomining technology are emerging disposal technologies that are helpful for the remediation of these hidden pollutants (hyperaccumulator plant harvests). Phytomining can be applied commercially, leading to additional environmental and economic benefits (Fu, et al., 2021).
5.2 Enzymatic and non-enzymatic antioxidant responses
One of the earliest biochemical changes that can occur when plants are exposed to heavy metals is an increase in ROS quantities, leading to oxidative damage (Dutta, et al., 2018; Kraj, et al., 2021). In response, the plant develops complicated mechanisms to adapt and survive under heavy metal stress. SOD activity in the roots and leaves of E. billardieri and A. rostratus was increased significantly compared to the control plants. The induction of the activity of SOD is one of the plants’ vital defense mechanisms, contributing to the detoxification of ROS. The upregulation of SOD and its induced activity play a critical role in plant survival by increasing the plant’s tolerance to stress conditions (Ahmad et al., 2010; Gill and Tuteja, 2010). The induced activity of SOD has been observed in other plants treated with heavy metals, reflecting the involvement of the antioxidant machinery in response to the toxicity of heavy metals (Ahmad, et al., 2010). For instance, it has been found that a low concentration of silver nanoparticles (1–20 mg/L) leads to a significant induction of the SOD activity in Solanum tuberosum, Wolffia globosa, Spirodela polyrhiza, and Lycopersicon esculentum (Yan and Chen, 2019). Induction of the SOD activity has also been reported in tall fescue plants treated with Cd, Cu, and As (Ahmad, et al., 2010). Moreover, there have been reports confirming the increases in the SOD gene expression in the plants treated with heavy metals (Espinosa-Vellarino, et al., 2020). These increases in SOD expression and activity, thus, prove the critical role of the enzyme in adapting to pollution caused by heavy metals.
CAT activity was induced notably in the leaves and roots of E. billardieri and A. rostratus grown in the mining area as compared to the control plants. Enhancement of the CAT activity is an adaptive behavior for overcoming stress (Ahmad, et al., 2010). Moreover, changes in gene expression encoding antioxidant enzymes or metal transporters are plants’ strategies for tolerating heavy metals (Skuza, et al., 2022). Over-expression of CAT and CAT3 genes in the root of Nicotiana tabacum in response to Cd contamination has also been previously confirmed (Gichner, et al., 2004). However, considering the excellent performance of the plants’ roots in the uptake and stabilization of the elements, as compared to their shoot, SOD and CAT in their root were at the highest level of activity. The vicinity of root to the soil pollutants could be another reason for the high activity of the enzymes in the root (Torbati, et al., 2021).
Total phenols and flavonoids in different parts of E. billardieri and A. rostratus were notably more than those in the control plants. Because of the ideal structural chemistry for free radical scavenging activity, the content of phenols and flavonoids is associated with ROS produced under stress conditions (Michalak, 2006). These compounds have been shown to be more effective free radical scavengers than other non-enzymatic antioxidants such as tocopherols and ascorbate. Phenolic compounds can also act as chelators of redox-active metal ions that are capable of catalyzing lipid peroxidation; therefore, they can mitigate the toxic effects of heavy metals by increasing the plant tolerance (Ahmad, et al., 2010). In agreement with the obtained results, it has been reported that the accumulation of Pb and Cu can result in an increase of the activity of phenylalanine ammonia-lyase (PAL) and tyrosine ammonia-lyase (TAL) enzymes at a concentration of 200 ppm (Jańczak-Pieniążek, et al., 2022). The mentioned TAL and PAL are the key enzymes involved in the activation of the phenylpropanoid pathway as a main source of phenols, flavonoids, and other non-enzymatic antioxidants production.
To conclude, the induction of the antioxidant enzyme activities and enhancement of the content of phenols and flavonoids in E. billardieri and A. rostratus are two main mechanisms used by the plants to tolerate heavy metal pollution in the mining area.
6 Conclusion
The present study was conducted to screen plants growing in the Zarshuran mining area to determine their potential for some metal accumulation. The pollution index’s measurement in the soil samples of the area confirmed that there was very intense enrichment of Ag, Cd, Pb, Zn, and Sb in the studied area. Among the 20 screened plant species, E. billardieri with ECR and ECS values more than 1 for Ag, Pb, Zn, and Cu elements had good potential in uptake, translocation, and phytostabilisation of the elements. A. rostratus with ECS and TF > 1 was determined as the plant species capable of Ag phytoextraction. Moreover, S. latifolia showed an acceptable ability to stabilize Ag, Pb, and Zn in its root. SOD activity and the amounts of total phenols and flavonoids in different parts of E. billardieri and A. rostratus were significantly more than those in the related control plants. Induction of the CAT activity in E. billardieri grown in the mining area was also confirmed. It seems that E. billardieri and A. rostratus counteract the heavy metal oxidative stress by inducing their antioxidant defense system. The capability of plant species to accumulate and tolerate heavy metals is a very important factor in the selection of the useful plants for phytoremediation.
Data availability statement
The original contributions presented in the study are included in the article/supplementary material, further inquiries can be directed to the corresponding authors.
Author contributions
ST: Writing–review and editing, Writing–original draft, Visualization, Validation, Supervision, Software, Resources, Project administration, Methodology, Investigation, Funding acquisition, Formal Analysis, Data curation, Conceptualization. SE: Writing–review and editing, Methodology, AA: Writing–review and editing, Validation, Methodology, Investigation, Formal Analysis.
Funding
The author(s) declare that financial support was received for the research, authorship, and/or publication of this article. The authors would like to express their gratitude to the Iran National Science Foundation (INSF) for supporting the present research under grant number 99019580.
Acknowledgments
The authors would also like to thank Urmia University for the support of the present study and Dr. Abbas Oruji for his cooperation in gathering the samples in the mining area.
Conflict of interest
The authors declare that the research was conducted in the absence of any commercial or financial relationships that could be construed as a potential conflict of interest.
The author(s) declared that they were an editorial board member of Frontiers, at the time of submission. This had no impact on the peer review process and the final decision.
Publisher’s note
All claims expressed in this article are solely those of the authors and do not necessarily represent those of their affiliated organizations, or those of the publisher, the editors and the reviewers. Any product that may be evaluated in this article, or claim that may be made by its manufacturer, is not guaranteed or endorsed by the publisher.
References
Ahmad, P., Jaleel, C. A., Salem, M. A., Nabi, G., and Sharma, S. (2010). Roles of enzymatic and nonenzymatic antioxidants in plants during abiotic stress. Crit. Rev. Biotechnol. 30, 161–175. doi:10.3109/07388550903524243
Aliyari, F., Afzal, P., and Abdollahi Sharif, J. (2017). Determination of geochemical anomalies and gold mineralized stages based on litho-geochemical data for Zarshuran Carlin-like gold deposit (NW Iran) utilizing multi-fractal modeling and stepwise factor analysis. J. Min. Environ. 8, 593–610. doi:10.22044/jme.2017.5252.1340
Aliyari, F., Yousefi, T., Abedini, A., and Calagari, A. A. (2021). Primary geochemical haloes and alteration zoning applied to gold exploration in the Zarshuran Carlin-type deposit, northwestern Iran. J. Geochem. Explor. 231, 106864. doi:10.1016/j.gexplo.2021.106864
Amin, H., Arain, B. A., Jahangir, T. M., Abbasi, M. S., and Amin, F. (2018). Accumulation and distribution of lead (Pb) in plant tissues of guar (Cyamopsis tetragonoloba L.) and sesame (Sesamum indicum L.): profitable phytoremediation with biofuel crops. Geol. Ecol. Landsc. 2, 51–60. doi:10.1080/24749508.2018.1452464
Anbuselvan, N., Senthil Nathan, D., and Sridharan, M. (2018). Heavy metal assessment in surface sediments off Coromandel Coast of India: implication on marine pollution. Mar. Pollut. Bull. 131, 712–726. doi:10.1016/j.marpolbul.2018.04.074
Anjum, N. A., Umar, S., and Iqbal, M. (2014). Assessment of cadmium accumulation, toxicity, and tolerance in Brassicaceae and Fabaceae plants—implications for phytoremediation. Environ. Sci. Pollut. Res. 21, 10286–10293. doi:10.1007/s11356-014-2889-5
Asadi, H. H., Voncken, J. H. L., Kühnel, R. A., and Hale, M. (2000). Petrography, mineralogy and geochemistry of the Zarshuran Carlin-like gold deposit, northwest Iran. Min. Deposita 35, 656–671. doi:10.1007/s001260050269
Azizi, M., Faz, A., Zornoza, R., Martinez-Martinez, S., and Acosta, J. A. (2023). Phytoremediation potential of native plant species in mine soils polluted by metal(loid)s and rare earth elements. Plants 12, 1219. doi:10.3390/plants12061219
Balafrej, H., Bogusz, D., Triqui, Z.-E. A., Guedira, A., Bendaou, N., Smouni, A., et al. (2020). Zinc hyperaccumulation in plants: a review. Plants 9, 562. doi:10.3390/plants9050562
Bastami, K. D., Bagheri, H., Haghparast, S., Soltani, F., Hamzehpoor, A., and Bastami, M. D. (2012). Geochemical and geo-statistical assessment of selected heavy metals in the surface sediments of the Gorgan Bay, Iran. Mar. Pollut. Bull. 64, 2877–2884. doi:10.1016/j.marpolbul.2012.08.015
Belimov, A. A., Malkov, N. V., Puhalsky, J. V., Tsyganov, V. E., Bodyagina, K. B., Safronova, V. I., et al. (2018). The crucial role of roots in increased cadmium-tolerance and Cd-accumulation in the pea mutant SGECdt. Biol. Plant. 62, 543–550. doi:10.1007/s10535-018-0789-0
Bhat, S. A., Bashir, O., Ul Haq, S. A., Amin, T., Rafiq, A., Ali, M., et al. (2022). Phytoremediation of heavy metals in soil and water: an eco-friendly, sustainable and multidisciplinary approach. Chemosphere 303, 134788. doi:10.1016/j.chemosphere.2022.134788
Bolan, N., Kumar, M., Singh, E., Kumar, A., Singh, L., Kumar, S., et al. (2022). Antimony contamination and its risk management in complex environmental settings: a review. Environ. Int. 158, 106908. doi:10.1016/j.envint.2021.106908
Chandra, S., Khan, S., Avula, B., Lata, H., Yang, M. H., ElSohly, M. A., et al. (2014). Assessment of total phenolic and flavonoid content, antioxidant properties, and yield of aeroponically and conventionally grown leafy vegetables and fruit crops: a comparative study. Evid. Based Complement. Altern. Med. 2014, 1–9. doi:10.1155/2014/253875
Collin, S., Baskar, A., Geevarghese, D. M., Ali, M. N. V. S., Bahubali, P., Choudhary, R., et al. (2022). Bioaccumulation of lead (Pb) and its effects in plants: a review. J. Hazard. Mater. Lett. 3, 100064. doi:10.1016/j.hazl.2022.100064
Cuevas, J., Faz, Á., Martínez-Martínez, S., Gabarrón, M., Beltrá, J., Martínez, J., et al. (2023). Bio-monitoring of metal(loid)s pollution in dry riverbeds affected by mining activity. Plants 12, 3775. doi:10.3390/plants12213775
Dutta, S., Mitra, M., Agarwal, P., Mahapatra, K., De, S., Sett, U., et al. (2018). Oxidative and genotoxic damages in plants in response to heavy metal stress and maintenance of genome stability. Plant Signal. Behav. 13, e1460048. doi:10.1080/15592324.2018.1460048
Egendorf, S. P., Groffman, P., Moore, G., and Cheng, Z. (2020). The limits of lead (Pb) phytoextraction and possibilities of phytostabilization in contaminated soil: a critical review. Int. J. Phytoremed. 22, 916–930. doi:10.1080/15226514.2020.1774501
Ehrampoush, M. H., Miria, M., Salmani, M. H., and Mahvi, A. H. (2015). Cadmium removal from aqueous solution by green synthesis iron oxide nanoparticles with tangerine peel extract. J. Environ. Health Sci. Eng. 13, 84–91. doi:10.1186/s40201-015-0237-4
Espinosa-Vellarino, F. L., Garrido, I., Ortega, A., Casimiro, I., and Espinosa, F. (2020). Effects of antimony on reactive oxygen and nitrogen species (ROS and RNS) and antioxidant mechanisms in tomato plants. Front. Plant Sci. 11, 674. doi:10.3389/fpls.2020.00674
Fu, Y., You, S., and Luo, X. (2021). A review on the status and development of hyperaccumulator harvests treatment technology. IOP Conf. Ser. Earth Environ. Sci. 634, 012113. doi:10.1088/1755-1315/634/1/012113
GhaderianGhotbi Ravandi, S. M. A. A. (2012). Accumulation of copper and other heavy metals by plants growing on Sarcheshmeh copper mining area, Iran. J. Geochem. Explor. 123, 25–32. doi:10.1016/j.gexplo.2012.06.022
Gichner, T., Patková, Z., Száková, J., and Demnerová, K. (2004). Cadmium induces DNA damage in tobacco roots, but no DNA damage, somatic mutations or homologous recombination in tobacco leaves. Mutat. Res. Genet. Toxicol. Environ. Mutagen. 559, 49–57. doi:10.1016/j.mrgentox.2003.12.008
GillTuteja, S. N. (2010). Reactive oxygen species and antioxidant machinery in abiotic stress tolerance in crop plants. Plant Physiol. biochem. 48, 909–930. doi:10.1016/j.plaphy.2010.08.016
Guda, M. A., Mutlag, N. H., and Tsear, A. A. (2020). The use of Atriplex nummularia plant as the hyperaccumulators of silver. AIP Conf. Proc. 2290, 020041. doi:10.1063/5.0027565
HarrisBali, A. T. R. (2008). On the formation and extent of uptake of silver nanoparticles by live plants. J. Nanopart. Res. 10, 691–695. doi:10.1007/s11051-007-9288-5
Honda, M., Tamura, H., Kimura, T., Kinoshita, T., Matsufuru, H., and Sato, T. (2007). Control of lead polluted leachate in a box-scale phytoremediation test using common buckwheat (Fagopyrum esculentum Moench) grown on lead contaminated soil. Environ. Technol. 28, 425–431. doi:10.1080/09593332808618805
Jańczak-Pieniążek, M., Cichoński, J., Michalik, P., and Chrzanowski, G. (2022). Effect of heavy metal stress on phenolic compounds accumulation in winter wheat plants. Molecules 28, 241. doi:10.3390/molecules28010241
Kabata-Pendias, A. (2010). Trace elements in soils and plants. 4th edn. Boca Raton, Florida: CRC Press, 505.
Karimi, N., Ghaderian, S. M., Maroofi, H., and Schat, H. (2009). Analysis of arsenic in soil and vegetation of a contaminated area in Zarshuran, Iran. Int. J. Phytoremed. 12, 159–173. doi:10.1080/15226510903213977
Kraj, W., Pietrzykowski, M., and Warczyk, A. (2021). “Handbook of bioremediation,” in Physiological, molecular and biotechnological interventions. Editors M. Hasanuzzaman, and M. N. V. Prasad (Academic Press), 205–220.
Li, C., Gao, T., Wang, X., Qu, S., Yang, Y., Zuo, M., et al. (2022). Phytoremediation potential and physiological mechanisms underlying metallic extraction of Suaeda glauca, Artemisia desertorum, and Atriplex canescens. Int. J. Environ. Res. Public Health 19, 16035. doi:10.3390/ijerph192316035
Lu, Y., Li, X., He, M., Zeng, F., and Li, X. (2017). Accumulation of heavy metals in native plants growing on mining-influenced sites in Jinchang: a typical industrial city (China). Environ. Earth Sci. 76, 446. doi:10.1007/s12665-017-6779-2
LuoZhang, J. S. Z. (2021). Mechanisms of cadmium phytoremediation and detoxification in plants. Crop J. 9, 521–529. doi:10.1016/j.cj.2021.02.001
Mansoor, S., Ali, A., Kour, N., Bornhorst, J., AlHarbi, K., Rinklebe, J., et al. (2023). Heavy metal induced oxidative stress mitigation and ROS scavenging in plants. Plants 12, 3003. doi:10.3390/plants12163003
Mehrabi, B., Yardley, B. W. D., and Cann, J. R. (1999). Sediment-hosted disseminated gold mineralisation at Zarshuran, NW Iran. Min. Deposita 34, 673–696. doi:10.1007/s001260050227
Michalak, A. (2006). Phenolic compounds and their antioxidant activity in plants growing under heavy metal stress. Pol. J. Environ. Stud. 15, 523–530.
Mishra, T., Pandey, V. C., Praveen, A., Singh, N. B., Singh, N., and Singh, D. P. (2020). Phytoremediation ability of naturally growing plant species on the electroplating wastewater-contaminated site. Environ. Geochem. Health 42, 4101–4111. doi:10.1007/s10653-020-00529-y
ModabberiMoore, S. F. (2004). Environmental geochemistry of Zarshuran Au-As deposit, NW Iran. Environ. Geol. 46, 796–807. doi:10.1007/s00254-004-1065-5
Napoli, M., Cecchi, S., Grassi, C., Baldi, A., Zanchi, C. A., and Orlandini, S. (2019). Phytoextraction of copper from a contaminated soil using arable and vegetable crops. Chemosphere 219, 122–129. doi:10.1016/j.chemosphere.2018.12.017
Obinger, C., Maj, M., Nicholls, P., and Loewen, P. (1997). Activity, peroxide compound formation, and heme d synthesis in Escherichia coliHPII catalase. Arch. Biochem. Biophys. 342, 58–67. doi:10.1006/abbi.1997.9988
Peer, W. A., Mahmoudian, M., Freeman, J. L., Lahner, B., Richards, E. L., Reeves, R. D., et al. (2006). Assessment of plants from the Brassicaceae family as genetic models for the study of nickel and zinc hyperaccumulation. New Phytol. 172, 248–260. doi:10.1111/j.1469-8137.2006.01820.x
Rajput, V. D., Harish, R. K., Verma, K. K., Sharma, L., Quiroz-Figueroa, F. R., Meena, M., et al. (2021). Recent developments in enzymatic antioxidant defence mechanism in plants with special reference to abiotic stress. Biology 10, 267. doi:10.3390/biology10040267
Rechinger, K. H. (1963). Flora Iranica. Vol. 1-175. Graz, Austeria: Akademische Druck und Verlagsanstalt.
Reeves, R. D., Baker, A. J. M., Jaffré, T., Erskine, P. D., Echevarria, G., and van der Ent, A. (2018). A global database for plants that hyperaccumulate metal and metalloid trace elements. New Phytol. 218, 407–411. doi:10.1111/nph.14907
Sachdev, S., Ansari, S. A., Ansari, M. I., Fujita, M., and Hasanuzzaman, M. (2021). Abiotic stress and reactive oxygen species: generation, signaling, and defense mechanisms. Antioxidants 10, 277. doi:10.3390/antiox10020277
Sarwar, N., Imran, M., Shaheen, M. R., Ishaque, W., Kamran, M. A., Matloob, A., et al. (2017). Phytoremediation strategies for soils contaminated with heavy metals: modifications and future perspectives. Chemosphere 171, 710–721. doi:10.1016/j.chemosphere.2016.12.116
Sasmaz, A. (2008). Translocation and accumulation of boron in roots and shoots of plants grown in soils of low boron concentration in Turkey's Keban Pb-Zn mining area. Int. J. Phytoremed. 10, 302–310. doi:10.1080/15226510802096119
ShahbaziBeheshti, K. M. (2019). Comparison of three methods for measuring heavy metals in calcareous soils of Iran. SN Appl. Sci. 1, 1541. doi:10.1007/s42452-019-1578-x
Shahid, M., Dumat, C., Khalid, S., Schreck, E., Xiong, T., and Niazi, N. K. (2017). Foliar heavy metal uptake, toxicity and detoxification in plants: a comparison of foliar and root metal uptake. J. Hazard. Mater. 325, 36–58. doi:10.1016/j.jhazmat.2016.11.063
Sheoran, V., Sheoran, A. S., and Poonia, P. (2016). Factors affecting phytoextraction: a review. Pedosphere 26, 148–166. doi:10.1016/S1002-0160(15)60032-7
Shtangeeva, I. (2021). About plant species potentially promising for phytoextraction of large amounts of toxic trace elements. Environ. Geochem. Health 43, 1689–1701. doi:10.1007/s10653-020-00633-z
Singleton, V. L., Orthofer, R., and Lamuela-Raventós, R. M. (1999). Methods in enzymology vol. 299. Academic Press, 152–178.
Skuza, L., Szućko-Kociuba, I., Filip, E., and Bożek, I. (2022). Natural molecular mechanisms of plant hyperaccumulation and hypertolerance towards heavy metals. Int. J. Mol. Sci. 23, 9335. doi:10.3390/ijms23169335
Sodhi, K. K., Mishra, L. C., Singh, C. K., and Kumar, M. (2022). Perspective on the heavy metal pollution and recent remediation strategies. Curr. Res. Microb. Sci. 3, 100166. doi:10.1016/j.crmicr.2022.100166
Stöcklin, J. (1968). Structural history and tectonics of Iran1: a review. AAPG Bull. 52, 1229–1258. doi:10.1306/5D25C4A5-16C1-11D7-8645000102C1865D
Tale Fazel, E., Pašava, J., Wilke, F. D. H., Oroji, A., and Andronikova, I. (2023). Source of gold and ore-forming processes in the Zarshuran gold deposit, NW Iran: insights from in situ elemental and sulfur isotopic compositions of pyrite, fluid inclusions, and O−H isotopes. Ore Geol. Rev. 156, 105382. doi:10.1016/j.oregeorev.2023.105382
Tang, H., Meng, G., Xiang, J., Mahmood, A., Xiang, G., Sana, Y., et al. (2022). Toxic effects of antimony in plants: reasons and remediation possibilities—a review and future prospects. Front. Plant Sci. 13, 1011945. doi:10.3389/fpls.2022.1011945
Taylor, S. R. (1964). Abundance of chemical elements in the continental crust: a new table. Geochim. Cosmochim. Acta 28, 1273–1285. doi:10.1016/0016-7037(64)90129-2
Torbati, S., Alipour, S., Rostami, M., and Hajializadeh, S. (2018). Bio-accumulation of major, trace, and rare earth elements by two Astragalus species grown on Agh-Dareh and Zarshouran gold deposits, Takab, NW Iran. J. Min. Environ. 9, 417–429. doi:10.22044/jme.2018.6995.1539
Torbati, S., Atashbar Kangarloei, B., and Khataee, A. (2021). Bioconcentration of heavy metals by three plant species growing in Golmarz wetland, in northwestern Iran: the plants antioxidant responses to metal pollutions. Environ. Technol. Inno. 24, 101804. doi:10.1016/j.eti.2021.101804
TorbatiEsmailbegi Kermani, S. S. (2022). Determining the concentration of Ag, Pb and Zn elements in some indigenous plant species grown in Zarshouran mining area, northwestern Iran. Iran. J. Health Environ. 15, 379–398.
Townsend, C. C., Guest, E., and Bor, N. L. (1966-1985). Flora of Iraq. Vol. 1-9. Baghdad, Iraq: Ministry of Agriculture and Agrarian Reform.
US EPA (2019). Method 3052 - microwave assisted acid digestion of siliceous and organically based matrices. Avaliable at: https://www.epa.gov/sites/production/files/2015-12/documents/3052.pdf.
Vidya, C. S.-N., Shetty, R., Vaculíková, M., and Vaculík, M. (2022). Antimony toxicity in soils and plants, and mechanisms of its alleviation. Environ. Exp. Bot. 202, 104996. doi:10.1016/j.envexpbot.2022.104996
Winterbourn, C. C., McGrath, B. M., and Carrell, R. W. (1976). Reactions involving superoxide and normal and unstable haemoglobins. Biochem. J. 155, 493–502. doi:10.1042/bj1550493
Worlanyo, A. S., and Jiangfeng, L. (2021). Evaluating the environmental and economic impact of mining for post-mined land restoration and land-use: a review. J. Environ. Manage. 279, 111623. doi:10.1016/j.jenvman.2020.111623
Yan, A., and Chen, Z. (2019). Impacts of silver nanoparticles on plants: a focus on the phytotoxicity and underlying mechanism. Int. J. Mol. Sci. 20, 1003. doi:10.3390/ijms20051003
Yan, Q., Fang, H., Wang, D., Xiao, X., Deng, T., Li, X., et al. (2023). Transfer and transformation characteristics of Zn and Cd in soil-rotation plant (Brassica napus L and Oryza sativa L) system and its influencing factors. Sci. Rep. 13, 7393. doi:10.1038/s41598-023-34377-4
Yoon, J., Cao, X., Zhou, Q., and Ma, L. Q. (2006). Accumulation of Pb, Cu, and Zn in native plants growing on a contaminated Florida site. Sci. Total Environ. 368, 456–464. doi:10.1016/j.scitotenv.2006.01.016
Zhang, Y., Song, B., Zhu, L., and Zhou, Z. (2021). Evaluation of the metal(loid)s phytoextraction potential of wild plants grown in three antimony mines in southern China. Int. J. Phytoremed. 23, 781–790. doi:10.1080/15226514.2020.1857685
Keywords: enrichment coefficients, heavy metal pollution, phytoremediation, phytostabilisation, Zarshuran gold deposit
Citation: Torbati S, Esmailbegi Kermani S and Abedini A (2024) Remediation of heavy metals by native plant species grown in Iran’s richest gold mine and study of plants’ pollution tolerance strategies. Front. Earth Sci. 12:1304497. doi: 10.3389/feart.2024.1304497
Received: 29 September 2023; Accepted: 22 February 2024;
Published: 21 March 2024.
Edited by:
Munesh Kumar, Hemwati Nandan Bahuguna Garhwal University, IndiaReviewed by:
Daniela Varrica, University of Palermo, ItalyBhupendra Singh, VCSG Uttarakhand University, India
Copyright © 2024 Torbati, Esmailbegi Kermani and Abedini. This is an open-access article distributed under the terms of the Creative Commons Attribution License (CC BY). The use, distribution or reproduction in other forums is permitted, provided the original author(s) and the copyright owner(s) are credited and that the original publication in this journal is cited, in accordance with accepted academic practice. No use, distribution or reproduction is permitted which does not comply with these terms.
*Correspondence: Samaneh Torbati, s.torbati@urmia.ac.ir; Ali Abedini, a.abedini@urmia.ac.ir