Spatiotemporal Variation on Fertility, Mating System, and Gene Flow in Vriesea gigantea (Bromeliaceae), an Atlantic Forest Species
- 1Institute of Bioscience, Federal University of Mato Grosso do Sul, Campo Grande, Brazil
- 2Department of Plant Biology, Institute of Biology, State University of Campinas, Campinas, Brazil
- 3National Institute of Agricultural Botany, Cambridge, United Kingdom
- 4Soil Genetics and Biology Laboratory, University of Santa Cruz do Sul, Santa Cruz do Sul, Brazil
- 5Departamento de Diagnóstico e Pesquisa Agropecuária, Secretaria da Agricultura, Pecuária e Desenvolvimento Rural–SEAPDR/RS, Porto Alegre, Brazil
- 6Department of Botany and Biodiversity Research, University of Vienna, Vienna, Austria
- 7Department of Genetics, Institute of Bioscience, Federal University of Rio Grande do Sul, Porto Alegre, Brazil
Knowledge of the patterns of variation in plant mating systems and fitness, in the context of conservation biology, is essential for understanding microscale differences and their probable causes. The aim of this study was to assess the patterns and determinants of spatiotemporal variation in fertility, mating systems, and gene flow in the epiphytic bromeliad Vriesea gigantea, which is endemic to the Atlantic Forest. We studied three wild populations over 2 years in terms of flowering, fruit set, seed production, and seed germination, and used nuclear microsatellite markers to analyse variations in the mating system and contemporary gene flow. We observed high fertility parameter values, with among-population differences only in fruit set and germination rate. Mating system analysis showed moderate outcrossing rates (tm), which ranged from 0.107 to 1.2, and strong pollen pool genetic structures (ΦFT), of 0.319 to 0.598 across populations and years. We suggest that the observed spatiotemporal variation in fertility, mating systems, and gene flow in this species are attributable to the adaptation to selfing due to edge effects, mainly caused by range expansion during post-glacial migration. Populations on the edge of the V. gigantea distribution were the most genetically divergent in the southern limit of the Atlantic Forest biome. Our results may contribute to ongoing debate regarding the characteristic fitness trade-offs that shape variation in plant mating systems and gene flow when species meet their range limits.
Introduction
Mating systems have important effects on the genetic diversity structure within and among populations in many flowering plants due to gene flow via pollen transference and seed dispersion. These factors directly affect the distribution of alleles and contribute to shaping the patterns of genetic diversity within and among populations (Hamrick, 1982; Loveless and Hamrick, 1984; Hamrick and Godt, 1996). Many studies on the reproductive ecology of plants have focused on field studies of reproductive systems and pollination aspects, along with the estimation of genetic and mating system parameters (Barrett and Eckert, 1990; Cascante-Marín et al., 2006; Eckert et al., 2009; Hmeljevski et al., 2011; Paggi et al., 2015). These studies provide valuable information regarding the spatial variation of mating systems and gene flow patterns in wild species (Moeller et al., 2017).
The outcrossing rate can be estimated by examining naturally-pollinated progeny arrays of plants. This approach excludes self-fertilised progeny from the data and uses a maximum-likelihood model (Ritland and Jain, 1981). In addition, conservation and evolutionary researchers concerned with different microevolutionary processes and the impact of landscape change must measure real-time gene movement. Austerlitz and Smouse (2001) and Smouse et al. (2001), developed a two-generation approach (TwoGener) to study pollen pool genetic structure, by quantifying the heterogeneity among the male gamete pools sampled by maternal plants distributed across populations, and estimating the mean pollination distance and effective neighbourhood size.
Genetic diversity and gene flow analyses are important for understanding the genetic diversity distribution of natural plant populations, which can influence local adaptation and provide valuable information for conservation decision-making (Segelbacher et al., 2021), although genetic diversity has often been neglected by environmental policy agreements (Hoban et al., 2021). Furthermore, genetic diversity is highly relevant to population viability, which can be reflected in survival and fecundity parameters determined by habitat conditions and biotic and abiotic factors (Reed and Frankham, 2003; Caruso et al., 2005; Leimu et al., 2006). Several fertility aspects have been studied to help estimate population viability, including plant size, flower production, fruit and seed set, and pollen and seed viability (Johnson et al., 2004; Paggi et al., 2007, 2013; Palma-Silva et al., 2008; Sampaio et al., 2012; Amat et al., 2013). Plant fruit and seed production can be influenced by the availability of biotic (pollen and pollinators; Barrett and Eckert, 1990; Colas et al., 2001; Johnson et al., 2004) and abiotic resources (Caruso et al., 2005). For example, pollen limitation on seed production can be a result of reduced pollinator visits or a decrease in the quantity or quality of pollen deposited per visit (Colas et al., 2001; Huang and Guo, 2002; Ashman et al., 2004; Buide, 2004; Ohara et al., 2006; Ratto et al., 2018; Bennett et al., 2020). In addition, fertility patterns associated with mating system analysis can provide a better understanding of biotic effects on the reproductive success of natural plant populations, especially when considering species from fragmented habitats (Aizen et al., 2002; Murren, 2002; Aguilar et al., 2006; Ohara et al., 2006), such as the Atlantic Forest (AF) located along the eastern coast of Brazil.
The relationship between plant fitness and patterns of genetic diversity differs between self-incompatible (positively correlated) and self-compatible (independent) species (Barrett, 2014). This relationship also affects population size and is a critical factor in plant ecology, evolution, and conservation. Even though many plant populations are naturally isolated and small, recent anthropogenic fragmentation of habitats has contributed to population reduction and isolation of several plant species (Leimu et al., 2006). In general, self-compatible species with large populations may experience less pronounced negative effects from habitat fragmentation (Leimu et al., 2006). Several studies have measured inbreeding depression, genetic variation, population size, and fitness of plant species to aid in their conservation (Stockwell et al., 2003; Kramer and Havens, 2009; Angeloni et al., 2011; Jacquemyn et al., 2012; Oakley and Winn, 2012; Frankham et al., 2014; Ottewell et al., 2016; Rodger et al., 2021).
Some species, such as epiphytic bromeliads live in stable, naturally fragmented habitats and are well-adapted to drought and wind, although their colonising ability is likely hindered by landscape structure, with only a limited number of suitable sites available (Benzing, 1990, 2000; Winkler et al., 2007; Victoriano-Romero et al., 2017). Bromeliad species frequently show high genetic differentiation among naturally fragmented populations, which could be a result of local adaptation by the species or genetic drift due to historical colonisation events (Zanella et al., 2012; Cascante-Marín et al., 2014; Hmeljevski et al., 2014; Goetze et al., 2016; Gonçalves-Oliveira et al., 2017; Godoy et al., 2018; Ruas et al., 2020; Vicente-Silva et al., 2021).
The Bromeliaceae family has approximately 3,140 described species (Butcher and Gouda, 2017), being among the most species-rich non-woody plant families in the Neotropics. It presents one of the most remarkable adaptive radiations in the plant world (Givnish et al., 2011, 2014). Around 50 percent of the species are epiphytic; they have evolved floral displays with a great diversity of colors, shapes, and scents that are related to pollinator attraction, with nectar being the usual reward (Zanella et al., 2012). These pollinators are bats, birds, and insects, in self- and outcrossing species; there are also autogamous taxa which do not need pollinator agents (Kessler and Krömer, 2000; Krömer and Kessler, 2006; Krömer et al., 2008; Kamke et al., 2011; Schmid et al., 2011a,b; Marques et al., 2015; Aguilar-Rodríguez et al., 2016). The species are also essential resource for small, hovering vertebrate pollinators in the New World (Fleming and Muchhala, 2008) and food sources for hummingbirds in many Neotropical forest regions (Dziedzioch et al., 2003; Wolowski et al., 2013; Pansarin and de Pedro, 2016; Nunes et al., 2018; Gomes et al., 2019; Lenzi and Paggi, 2020).
In the present study, we investigated patterns of spatiotemporal variation in mating systems and fertility of three populations of Vriesea gigantea Gaudichaud from the center of the range of the species to the southern margin over 2 years. Vriesea gigantea is a self-compatible species which showed high fertility parameters in one population evaluated (Paggi et al., 2007; Palma-Silva et al., 2008). Paggi et al. (2015) reported a high selfing rate (s = 0.612), and Sampaio et al. (2012) observed moderate to low inbreeding depression (δ = 0.02–0.39) in populations from its southern range margin. In addition to the reported high fertility, pollen limitation was observed due to habitat fragmentation (Paggi et al., 2007), although low pollen viability in some localities could also contribute to this effect (Palma-Silva et al., 2008). Palma-Silva et al. (2009) observed a latitudinal trend of decreasing genetic diversity from north to south away from the equator, consistent with the prediction of declining within-population and/or increasing among-population genetic diversity toward range margins (Eckert et al., 2008). For V. gigantea, Palma-Silva et al. (2009) hypothesised that the observed results are in concordance with the direction of historical forest expansion after the Last Maximum Glacial and the lack of gene flow at the current northern and southern range edges, which is partially explained by restricted seed dispersal (Paggi et al., 2010).
The focus of the present study was to evaluate the potential of plants to evolve local mating system adaptations, such as selfing, in relation to environmental changes (Eckert et al., 2010), for instance, edge effects and habitat loss. Specifically, we used our combined fertility and mating system data across populations and years to address the following questions: (1) Is there spatiotemporal variation in fertility and mating system parameters, and if so, how is this variation distributed in space and time? (2) Is there any correlation between spatiotemporal variation in outcrossing rates and fertility parameters? (3) What do estimates of the pollen pool genetic structure and the effective number of pollen donors tell us about gene flow among populations in different years?
Materials and Methods
Study Species and Sites
Vriesea gigantea is an epiphytic bromeliad species (occasionally lithophytic), which is endemic to the AF and occurs from the Espírito Santo to the Rio Grande do Sul states, Brazil (Figures 1B,E,F,J; Zimmermann et al., 2007; Palma-Silva et al., 2009; Paggi et al., 2015). This species has been described as being bat-pollinated (Sazima et al., 1999), but hummingbirds and bees may also play important roles in its pollination (Paggi et al., 2021), as also observed for Billbergia horrida Regel (Marques et al., 2015) and Tillandsia heterophylla E. Morren (Aguilar-Rodríguez et al., 2016). Typical inflorescences of V. gigantea have a central axis with several branches on each side and one flower on each side of the lateral axis (Reitz, 1983). Flowers at the base and centre of the inflorescence open first, followed by flowers in the apical position (Reitz, 1983; Benzing, 2000; Paggi et al., 2021). Anthesis may occur during both the day and night (Paggi et al., 2021). The flowers are tube-shaped with three petals, and their yellowish colour corresponds to the chiropterophilous syndrome (Figure 1G; Paggi et al., 2021), suggesting that outcrossing is the predominant mating system of this species (Vogel, 1969).
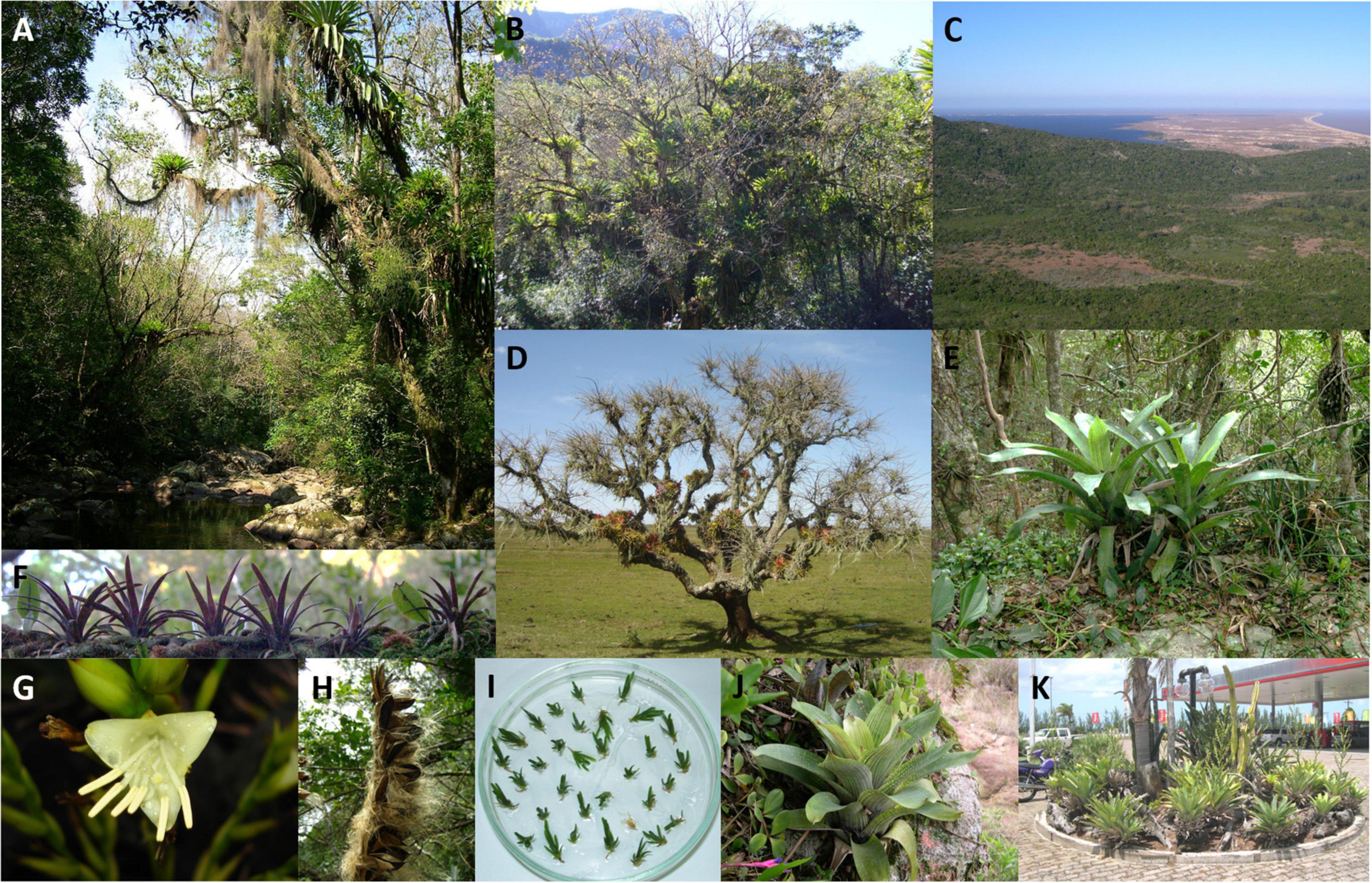
Figure 1. General view of Vrisea gigantea natural populations from Rio Grande do Sul state, Brazil, and details of the species. (A) Epiphytic individuals of V. gigantea in the Maquiné populations, showing individuals of Tillandsia usneoides (L.) Nutt. ex Steud., (B) Serra Geral Ecological Reserve overview, Maquiné city population, with several epiphytic individuals of V. gigantea, (C) Itapuã State Park overview, Porto Alegre city, (D) Taim Ecological Station, Rio Grande city, in detail an individual of Erythrina crista-galli L. Var. hasskarlii Backer with several epiphytic individuals of Aechmea recurvata (Klotzsch) L.B. Smith, several individuals Ramalina usnea (L.) R. Howe, and some individuals of Usnea sp. Dill. ex. Adans. (E) Lithophytic adult individual of V. gigantea, Itapuã population, (F) Epiphytic seedlings of V. gigantea, Itapuã population, (G) Flower detail of V. gigantea, Maquiné population, (H) Detail of seed dispersal of V. gigantea, Itapuã population, (I) V. gigantea seedlings from seed germinations experiments, (J) Lithophytic young individual of V. gigantea, Itapuã population, (K) Adult individuals of V. gigantea used for ornamental purposes at a gas station, BR 101, Maquiné city. Photographs: Clarisse Palma-Silva and Gecele Matos Paggi.
We conducted the present study with three wild populations of V. gigantea, Maquiné, Itapuã, and Taim, which were previously evaluated for genetic, mating system and fitness parameters in one reproductive year (Paggi et al., 2007, 2015; Palma-Silva et al., 2008, 2009; Sampaio et al., 2012). We conducted fieldwork on all populations during the flowering and fruiting periods of 2006 and 2007. Maquiné and Itapuã populations are located in the centre of the distribution of the species (Atlantic Forest strict sense), and Taim population at the range margin (Atlantic Forest wide sense), east of Rio Grande do Sul state, southern Brazil [Table 1 and Figures 1A–D; for more details see Paggi et al. (2015)]. These populations comprise a large number of individuals, which are in or close to environmental protection areas [Table 1 and Figure 1B; for more details see Paggi et al. (2015)], although the species is continually threatened by habitat loss and illegal predatory gathering (Table 1 and Figure 1K). The climate in the study region is classified as humid subtropical (Cfa) by the Köppen Climate Classification System.
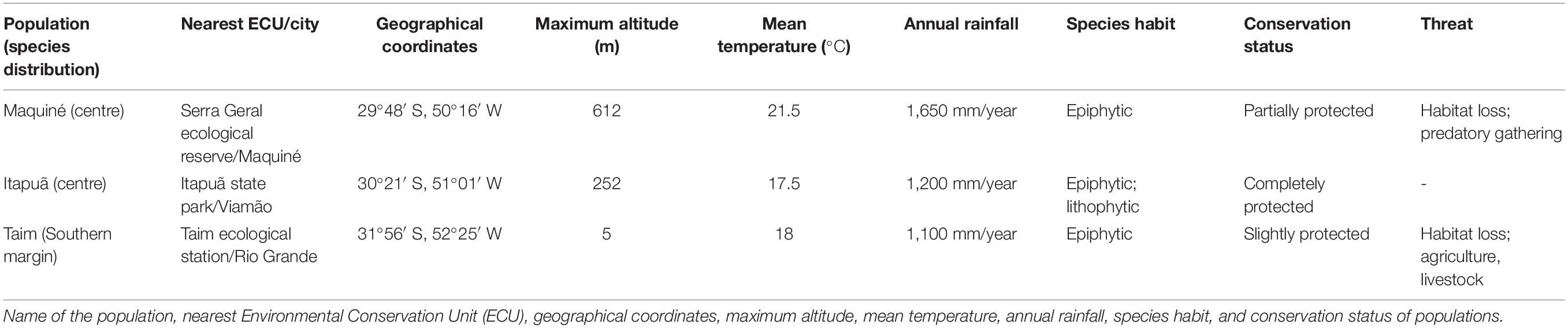
Table 1. Natural populations of Vriesea gigantea in the Atlantic Forest, Rio Grande do Sul State, Brazil.
Fertility Analysis
To evaluate the fertility of each population, we computed the following parameters using different individuals for each year (Table 2): (a) total number of flowers per plant; (b) total fruit set (i.e., the fraction of flowers developing into mature fruit) per plant; and (c) number of seeds per fruit (from one fruit randomly collected from 25 plants in each population, n = 75, Figure 1H). To analyse the seed germination rate, we used two fruits and 30 seeds per fruit per sampled individual (Table 2, n = 60 seeds per individual, Figure 1I). We germinated and monitored seeds daily for 30 days following the methods described by Paggi et al. (2007). We analysed fertility parameters by analysis of variance (ANOVA) followed by Tukey’s test to verify differences among populations and years. We tested for possible correlations between the outcrossing rate (tm) and fruit set considering all populations and years. We performed all statistical analyses using SAS software (Version 8, SAS Institute, Cary, NC, United States).
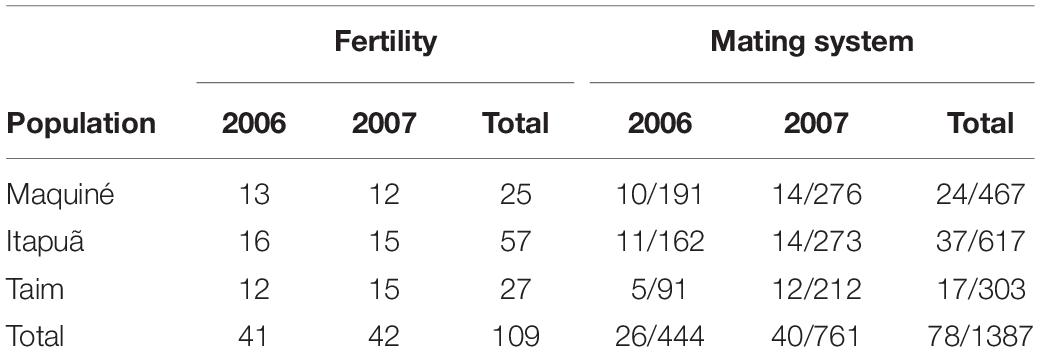
Table 2. Sample sizes per population and year for fertility (adult plants) and mating system analysis (adult plants/progenies) in the three studied populations of Vriesea gigantea.
Mating System Analysis
For genetic analysis, we collected open-pollinated seeds from different mother plants (MPs) each year (Table 2) because this species exhibits a semelparous life cycle, which means that an adult plant only blooms once in a lifetime. From each MP, we collected fresh leaves both in the field (∼5 cm2) and from seedlings germinated in vitro. The leaves were stored in liquid nitrogen until DNA extraction. Total genomic DNA was extracted according to the protocol described by Doyle and Dickson (1987). Using a global positioning system (GPS) receiver, we recorded the location of each MP. The total sample size, considering MPs and seedlings from all years and populations, was 1,465 individuals.
We genotyped four microsatellite loci for data analysis from 2006 and scored three nuclear microsatellite loci previously characterised for V. gigantea, VgB06, VgF01, and VgG02 (Palma-Silva et al., 2007); and one locus characterised for T. fasciculata, CT5 (Boneh et al., 2003). For the data analysis from 2007, we genotyped two of the most polymorphic loci identified in previous analyses (loci: VgF01 and VgG02), due to the occurrence of monomorphic loci in some sampled populations in the previous year. We labelled the forward primer of each locus at the 5’ end with an M13 fluorescent tag (6-FAM). We performed all polymerase chain reaction (PCR) amplifications in a PE Applied Biosystems 9700 thermocycler as described by Palma-Silva et al. (2007). Microsatellite alleles were resolved on a 3100 DNA Analyser (Applied Biosystems, Foster, United States) and precisely sized against an ROX molecular size standard using Genotyper 3.7 software (Applied Biosystems, Foster, United States).
We performed progeny pair analysis using the mixed mating model described by Ritland (2002) with Mltr 3.4 software (Ritland, 1990). We estimated the outcrossing rate (tm), inbreeding coefficient of maternal parents (F), correlation of selfing (rs) (Ritland, 2002), correlation of outcrossed paternity (rp; which reflects any tendency for siblings to share the same male parent) (Ritland, 2002), and number of effective pollen donors (Nep = 1/rp). We calculated standard errors based on 1,000 bootstraps between individuals within a progeny array. Using one-tailed Student’s t-tests, we determined the significance of tm and F based on the null hypothesis that tm = 1 and F = 0 using SAS software.
To analyse the genetic structure of pollen pools (ΦFT) of individual plants relative to the global pollen pool (Smouse et al., 2001) we used the TwoGener procedure, based on the AMOVA approach described by Excoffier et al. (1992). We computed the 99% confidence interval of ΦFT by bootstrapping among the loci with 1,000 replicates. We performed computations using the POLDISP 1.0 software (Robledo-Arnuncio et al., 2007) to estimate the pollen pool structure (ΦFT), average distance of pollination (δ), effective neighbourhood pollination area (Aep), and effective number of pollen donors (Nep).
Results
Fertility
The mean number of flowers per plant for all populations and years was 169.2 (standard error [SE] ± 5.6), with a range of 154.1 (SE ± 11.3, Itapuã–2006) to 201.2 (SE ± 20.7, Maquiné–2006), and no statistical differences were observed among populations (Table 3). The fruit set per plant ranged from 23.3% (SE ± 6.0, Itapuã–2006) to 75.9% (SE ± 5.6, Taim–2006) with an overall mean of 47.6% (SE ± 2.5), across all populations and years. We detected differences in fruit set among the populations in each year. The Taim population yielded more fruits in 2006 (75.9 ± 5.6), while the Itapuã population showed a lower fruit set in 2006 (23.3% ± 6.0; Table 3). The mean number of seeds per fruit was 598.4 (SE ± 35.2), and there were no differences among the populations (Table 3). We evaluated seed viability based on the germination rate of V. gigantea, which was high in all the populations. After 15 days of culture, an average of 86.8% (SE ± 2.5) of the seeds germinated (Figure 1I). The Taim population had the lowest germination rate of 73.0% (SE ± 6.3; Table 3).
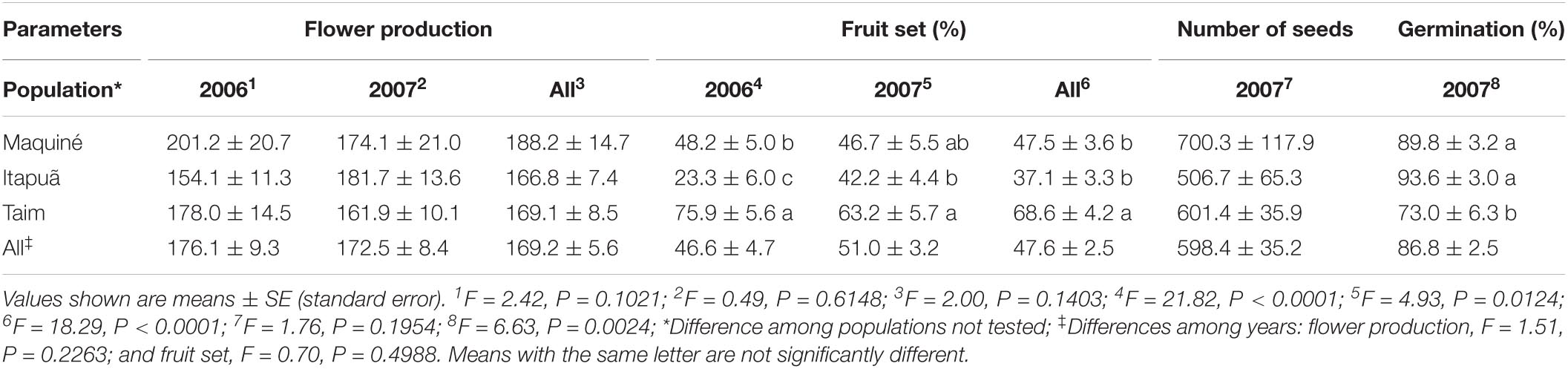
Table 3. Fertility parameters, including mean number of seeds per fruit, germination rate (n = 30 seeds per fruit), mean number of flowers per plant, and fruit set of Vriesea gigantea, in the three studied populations and the 2 years evaluated.
Mating System
The inbreeding coefficient (F) based on the genotypic frequencies of maternal plants was positive and significantly higher than zero in all populations and years (Table 4, except in Taim–2007), suggesting an excess of homozygotes (P < 0.0001). The outcrossing rate (tm) was variable among populations as well as between years within populations, ranging from 0.107 (Itapuã–2007) to 0.445 (Maquiné–2006; Table 4). A significant self-fertilisation rate (s = 1−tm) was detected since the tm was significantly lower than one for all populations and years (P < 0.0001). The correlation of selfing (rs) was high in the Maquiné population, implying that some families were highly selfed and others were highly outcrossed (Table 4). In the other populations, the rs values were lower, indicating less variation in selfing among the families. The correlation of the outcrossed paternity (rp) ranged from 0.286 (Itapuã–2007) to 0.714 (Maquiné–2006), suggesting that a high proportion of siblings shared the same male parent. Patterns of fruit set observed in V. gigantea populations were not significantly correlated with outcrossing rates; however, a slight increase in fruit set was observed when there was an increased outcrossing rate (R = 0.2742, P = 0.5518; Figure 2).
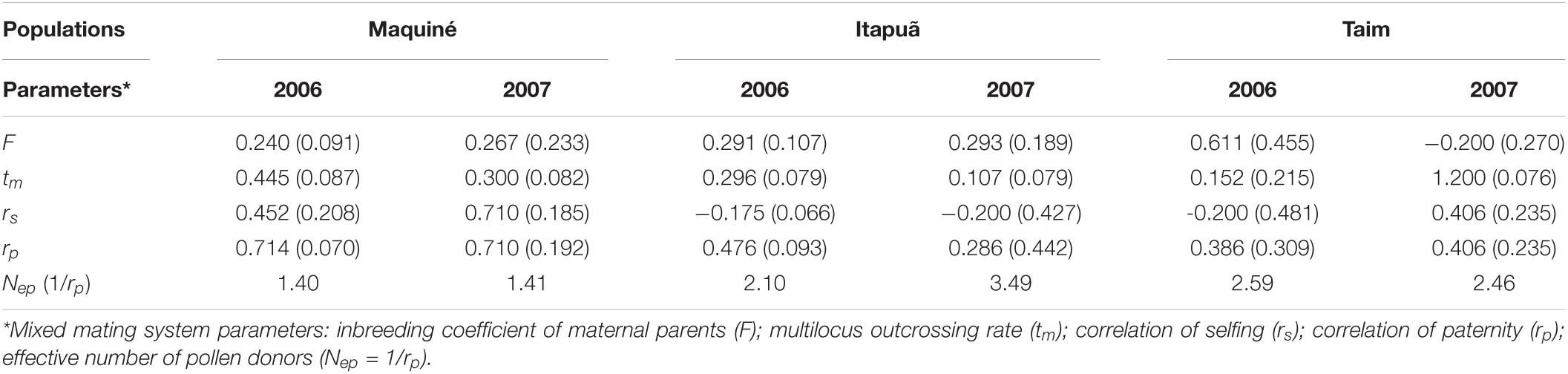
Table 4. Mating system parameters (SD—standard deviation in parentheses) of Vriesea gigantea populations and years.
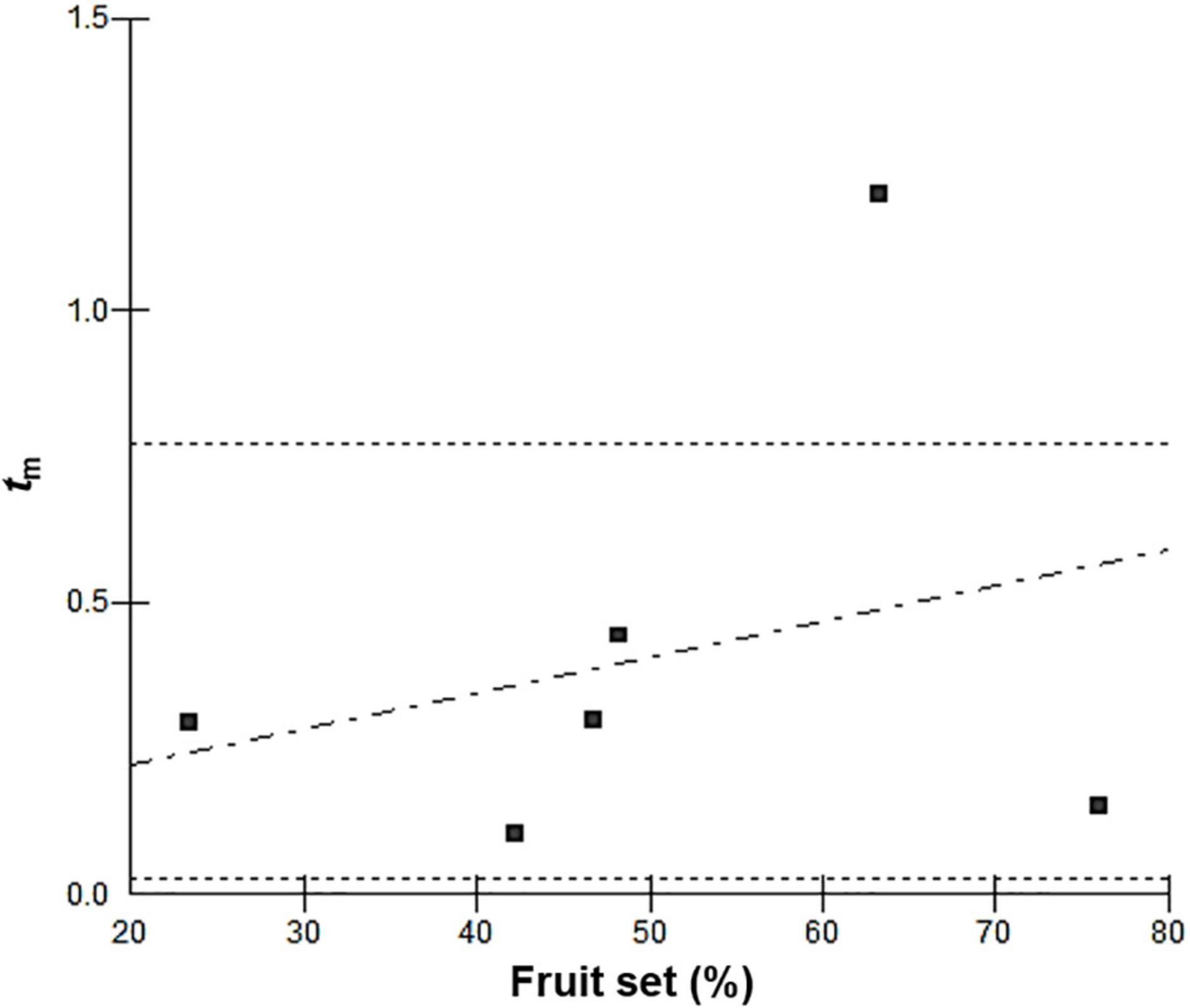
Figure 2. Correlation between fruit set and outcrossing rate in Vriesea gigantea (r = 0.2742, P < 0.5518) for populations of Maquiné/2006, Maquiné/2007, Itapuã/2006, Itapuã/2007, Taim/2006, and Taim/2007, which were plotted in this order in the graphic. The horizontal dotted line delimits the boundary of one standard deviation.
The differentiation in allelic frequencies among pollen pools (ΦFT) of V. gigantea ranged from 0.319 to 0.598, indicating differences in the distribution of pollen pools from plant to plant across populations and years (Table 5). The average pollination distance (δ) ranged from 77 to 299 m, and the effective neighbourhood pollination area (Aep) varied from 47 to 718 m2. The lowest average pollination distance and effective neighbourhood pollination area were observed in Itapuã–2007 when reproductive adult density was highest (17.4 plants/km2; data not shown). The effective number of pollen donors (Nep) for all populations and years was low, ranging from 0.77 to 1.56 (Table 5), with higher values in the more central population (Maquiné). We could not compute the estimates for Taim–2007, since the loci were monomorphic. The mating system parameters exhibited a notable trend from the range centre of the species (Maquiné) toward the southern range margin (Taim), with an increase in the inbreeding coefficient (F) and pollen pool genetic structure (ΦFT), and a decrease in the outcrossing rate (tm) (Figure 3).
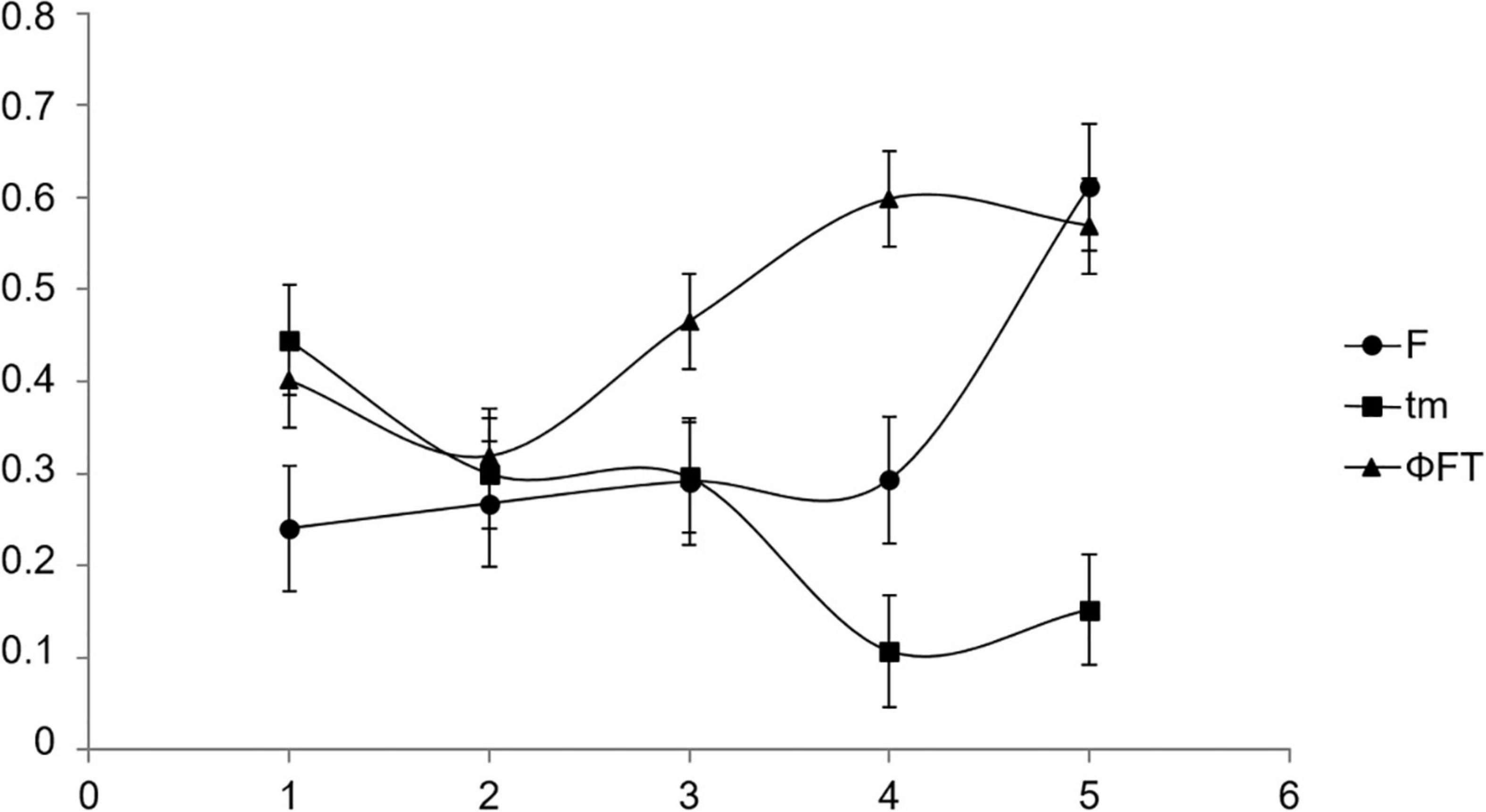
Figure 3. Variation (means ± SD) in inbreeding coefficients (F; circles), outcrossing rates (tm, squares), and pollen pool genetic structure (ΦFT, triangles), for populations from North to South of the geographic distribution of Vriesea gigantea: 1-Maquiné/2006; 2-Maquiné/2007; 3-Itapuã/2006; 4-Itapuã/2007; 5-Taim/2006.
Discussion
In this study, we observed spatiotemporal variation in fertility, mating systems, and gene flow of wild populations of V. gigantea from the centre to the southern range edge of the species. Unfortunately, we found no populations in geographically intermediate localities among the studied populations. No significant differences were observed when comparing temporal variations. Nevertheless, spatial variation was observed from the range centre of the species (north) toward the southern range margin (south), with an evident trend of increasing pollen pool genetic structure, decreasing outcrossing rate and no apparent changes in fertility parameters with latitude. The sampled population in the extreme southern edge of the distribution (Taim) exhibited the highest selfing rate and strongest pollen pool genetic structure. The results presented here are important for ongoing debate regarding the cost of and evolutionary significance of the adaptation to selfing in plant mating systems.
Across populations and years, mating systems and fertility analyses revealed that the studied populations had both high selfing rates and high fertility values. We observed a large production of flowers, fruits, and seeds, and high seed germination rates in all populations. Nevertheless, a more detailed examination of the results revealed that each population behaved differently in terms of the mating system and fertility. Although the Itapuã and Maquiné populations had the highest tm values, they showed the lowest fruit production, which could indicate pollen limitation due to ecological factors (Knight et al., 2005). In this case, qualitative (pollen viability) and/or quantitative (pollinator limitation) components, which had been previously identified in the Itapuã population as factors that decrease reproductive fitness, could be responsible for the observed results (Paggi et al., 2007; Palma-Silva et al., 2008).
Both populations showed high germination rates consistent with the low levels of inbreeding depression previously observed [δ = 0.05 in Maquiné, and δ = 0.03 in Itapuã; Sampaio et al. (2012)]. This result may indicate a significant contribution of outcrossing, although some unavoidable geitonogamy is expected to occur in self-compatible species that have stable low-to-intermediate selfing rates (Schoen and Lloyd, 1992; Lloyd, 1992; Porcher and Lande, 2005; Paggi et al., 2007, 2015). In contrast, the Taim population showed the highest fruit set, probably due to autonomous selfing or geitonogamy (Paggi et al., 2015). The results also indicated low cross-pollen competition, since we observed a high proportion of selfing (s = 0.848). Together, these features should effectively provide reproductive assurance for the species (Lloyd, 1992; Lloyd and Schoen, 1992); however, the Taim population had the lowest germination rate, likely due to inbreeding depression (δ = 0.02; Sampaio et al., 2012). Although we used a limited number of SSR loci to estimate these parameters, Palma-Silva et al. (2009) reported very similar results of low genetic diversity levels for Taim population (HO = 0.039 and FIS = 0.408) using ten SSR loci.
Variations in outcrossing rates across time and space can be attributed to the historical and biological contexts of each plant species (Loveless and Hamrick, 1984; Schemeske and Lande, 1985; Barrett and Eckert, 1990; Knight et al., 2005; Moeller et al., 2017). Most of this variation occurs in self-compatible species in which environmental and demographic factors can play essential roles in the degree of outcrossing due to differences in pollen quality and pollen vectors (Barrett and Eckert, 1990; Barrett, 2014). Those reproductive outcomes can vary among populations of animal-pollinated plants owing to differences in the availability of effective pollinators and environmental effects on plant phenotypes and their functional consequences for pollinator behavior that governs pollen dispersal (Tian et al., 2022). Here, we observed spatial inter-population variation in the mating system of V. gigantea, with an increase in selfing rates from north to south, and different effects on fertility parameters that can occur due to ecological or genetic factors (Barrett, 2014). Previous studies have shown that this species is self-compatible (Paggi et al., 2007) with some level of autonomous selfing and geitonogamy, although it also presents a cryptic self-incompatibility system with delayed germination of self-pollen at the stigma (Paggi et al., 2015). Moreover, Paggi et al. (2021) showed that hummingbirds are effective pollinators in the Southern populations of V. gigantea, even though the species is classified as chiropterophilous; the authors also reported only one hummingbird species for Itapuã and Taim populations, which may be related to the high levels of selfing observed here for both populations (Paggi et al., 2021). Additionally, low-to-moderate levels of inbreeding depression have been observed in the same population (Sampaio et al., 2012). Together, these features corroborate our observations of spatio-interpopulation variation in mating systems and fertility for the studied populations.
Results from the progeny analysis confirmed that selfing occurs at a moderate to high frequency (0.555 < s < 0.893; excluding data from Taim–2007), apparently as a consequence of different modes of self-fertilisation that contribute to reproductive assurance in V. gigantea populations when outcrossing is not possible. Many functional, ecological, morphological, and physiological factors affect the occurrence of self-fertilisation (Lloyd and Schoen, 1992). In the case of V. gigantea, this is more likely due to pollen limitation (lack of pollinator activity or low pollen quality; Paggi et al., 2007, 2021; Palma-Silva et al., 2008); however, demographic factors, such as genetic drift and consequently low genetic diversity could affect populations at the southern range margin, which have the highest selfing rates, and where natural populations have become scarce (personal observation). In many models for the selection of self-fertilisation, pollen limitation has been reported to be responsible for stable mixed mating systems with high selfing rates (Lloyd, 1992; Porcher and Lande, 2005), as was observed with V. gigantea. Models of mating system evolution that combine functional and genetic components have demonstrated that intermediate selfing is optimal for achieving the greatest possible fertility, implying that partial selfing may be adaptive and evolutionarily stable (Johnston et al., 2009). However, our results showed a slight positive correlation between outcrossing rates and fruit set in V. gigantea (Figure 2), indicating that outcrossing rates still have a significant influence on the fertility of this species, in which we observed lower fitness (germination rate) when high selfing rates occurred (Taim population, Tables 3, 4).
Fragmented habitats have a profound effect on the gene flow, mating patterns, and fertility of natural plant populations (Keller and Waller, 2002; Lienert, 2004; Aguilar et al., 2008). Previous molecular studies have indicated that V. gigantea populations at the margin of the species distribution range exhibit increased genetic drift due to reduced gene flow (Nem = 0.688) among populations, and present smaller effective population sizes (Ne) when compared to central populations located in the continuous forest (Palma-Silva et al., 2009). Additionally, we observed spatial inter-population variation in the outcrossing rates of V. gigantea, from low to moderate (south 0.107 < tm < 0.445 north; Table 4 and Figure 3), and high levels of pollen pool structure (north 0.319 < ΦFT < 0.598 south; Table 5 and Figure 3). These results corroborate the low levels of gene flow among populations from the southern range margin reported by Palma-Silva et al. (2009).
The fertility parameters revealed that, even with low gene flow, low effective population size (Palma-Silva et al., 2009), moderate inbreeding depression (Sampaio et al., 2012), strong pollen pool structure, and low effective number of pollen donors, all populations had high fitness levels (with high levels of fruit set and viable seed production). These results suggest that the observed among-population patterns of fertility, mating systems, and gene flow in V. gigantea may be attributable to adaptation for selfing, likely due to edge effects (Baker, 1955; Sun and Ritland, 1998; Pannell et al., 2015) during range expansion (during post-glacial migration; Palma-Silva et al., 2009; Moeller et al., 2017). However, other adaptive or non-adaptive casual factors cannot be excluded, such as pollinator behaviour or local densities of flowering plants.
Conclusion
By examining the spatiotemporal patterns of fertility, mating system, and gene flow parameters for all populations and years, we showed that V. gigantea presented a combination of high fertility, high selfing rate, and low gene flow among populations with a geographic distance higher than the average distance of pollination, which also resulted in a strong pollen pool structure. The Atlantic Forest is experiencing rapid ongoing fragmentation, and bottlenecks and low gene flow are expected to contribute to increasing genetic differentiation among populations in addition to high selfing rates. Considering the observed spatiotemporal variation, V. gigantea populations from the range edge, such as, the Taim population, should be granted conservation priority, since most of V. gigantea individuals occurs outside the conservation unit, and are threatened by habitat loss due to agriculture and livestock actions. Conservation strategies that protect populations at the range edge of the species may contribute to the continued survival and maintenance of the evolutionary potential and local adaptation of this species.
Data Availability Statement
The original contributions presented in this study are included in the article/supplementary material, further inquiries can be directed to the corresponding author.
Author Contributions
GP and FB designed the study. GP, CP-S, CZ, MG, and MB collected the material, performed the experiments, and analyzed the data. GP, CL, and FB wrote and reviewed the manuscript. All authors contributed to the article and approved the submitted version.
Funding
This work was supported by the National Council of Technological and Scientific Development—CNPq; Pró-Reitoria de Pesquisa da Universidade Federal do Rio Grande do Sul—PROPESQ/UFRGS; Programa de Pós-Graduação em Genética e Biologia Molecular—PPGBM/UFGRS; Kew Latin-American Research Fellowship Program—KLARF, from Andrew W. Mellon Foundation; and the International Foundation of Science—IFS. FB, CP, and GP were granted by a CNPq fellowship (numbers 309517/2015-0, 305398/2019-9, and 301596/2015-8, respectively). This study was financed in part by the Coordenação de Aperfeiçoamento de Pessoal de Nível Superior—Brasil (CAPES)—Finance Code 001.
Conflict of Interest
The authors declare that the research was conducted in the absence of any commercial or financial relationships that could be construed as a potential conflict of interest.
Publisher’s Note
All claims expressed in this article are solely those of the authors and do not necessarily represent those of their affiliated organizations, or those of the publisher, the editors and the reviewers. Any product that may be evaluated in this article, or claim that may be made by its manufacturer, is not guaranteed or endorsed by the publisher.
Acknowledgments
We thank Thelma Barbará, Eliane Kaltchuk-Santos, Tânia Wendt, Maurício Sedrez dos Reis, and Tatiana Souza-Chies for helpful discussions. We also thank Adriele Guerra, Guilherme Chazan, Jamilla Alves Trindade Sampaio, Lucas Caetano Tieppo da Silveira, and Manuela Bruxel for help with sample collection, fieldwork, and DNA extractions. We also thank the Itapuã State Park and Taim Ecological Station for logistical support, especially to Mr. Jairo Soares for the fieldwork support. We also thank IBAMA (Instituto Brasileiro do Meio Ambiente e dos Recursos Naturais Renováveis) and SEMA (Secretaria do Meio Ambiente e Infraestrutura, Rio Grande do Sul state) for the processing of collection permits. We also thank Editage (www.editage.com) for English language editing.
References
Aguilar, R., Ashworth, L., Galetto, L., and Aizen, M. A. (2006). Plant reproductive susceptibility to habitat fragmentation: review and synthesis through a meta-analysis. Ecol. Lett. 9, 968–980. doi: 10.1111/j.1461-0248.2006.00927.x
Aguilar, R., Quesada, M., Ashworth, L., Herrerias-Diego, Y., and Lobo, J. (2008). Genetic consequences of habitat fragmentation in plant populations: susceptible signals in plant traits and methodological approaches. Mol. Ecol. 17, 5177–5188. doi: 10.1111/j.1365-294X.2008.03971.x
Aguilar-Rodríguez, P. A., Krömer, T., García-Franco, J. G., and MacSwiney, G. M. C. (2016). From dusk till dawn: nocturnal and diurnal pollination in the epiphyte Tillandsia heterophylla (Bromeliaceae). Plant Biol. 18, 37–45. doi: 10.1111/plb.12319
Aizen, M. A., Ashworth, L., and Galetto, L. (2002). Reproductive success in fragmented habitats: do compatibility systems and pollination specialization matter? J. Veg. Sci. 13, 885–892.
Amat, M. E., Silvertown, J., and Vargas, P. (2013). Strong Spatial Genetic Structure Reduces Reproductive Success in the Critically Endangered Plant Genus Pseudomisopates. J. Hered. 104, 692–703. doi: 10.1093/jhered/est042
Angeloni, F., Ouborga, N. J., and Leimu, R. (2011). Meta-analysis on the association of population size and life history with inbreeding depression in plants. Biol. Conserv. 144, 35–43. doi: 10.1111/j.0014-3820.2003.tb00309.x
Ashman, T.-L., Knight, T. M., Steets, J. A., Amarasekare, P., Burd, M., Campbell, D. R., et al. (2004). Pollen limitation of plant reproduction: ecological and evolutionary causes and consequences. Ecology 85, 2408–2421.
Austerlitz, F., and Smouse, P. E. (2001). Two-Generation Analysis of Pollen Flow Across a Landscape. II. Relation Between ΦFT, Pollen Dispersal and Interfemale Distance. Genetics 157, 851–857. doi: 10.1093/genetics/157.2.851
Baker, H. G. (1955). Self-compatibility and establishment after “long-distance” dispersal. Evolution 21, 219–238.
Barrett, S. C. H. (2014). “Evolution of mating systems: outcrossing versus selfing,” in The Princeton Guide to Evolution, ed. J. Losos (Princeton, NJ: Princeton University Press), 356–362.
Barrett, S. C. H., and Eckert, C. G. (1990). “Variation and evolution of mating systems in seed plants,” in Biological Approaches and Evolutionary Trends in Plants, ed. S. Kawano (Tokyo: Academic Press), 229–254.
Bennett, J. M., Steets, J. A., Burns, J. H., Burkle, L. A., Vamosi, J. C., Wolowski, M., et al. (2020). Land use and pollinator dependency drives global patterns of pollen limitation in the Anthropocene. Nat. Commun. 11:3999. doi: 10.1038/s41467-020-17751-y
Benzing, D. H. (2000). Bromeliaceae: Profile of an Adaptive Radiation. Cambridge, UK: Cambridge University Press.
Boneh, L., Kuperus, P., and Van Tienderen, P. H. (2003). Microsatellites in bromeliads Tillandsia faciculata and Guzmania monostachya. Mol. Ecol. Notes 3, 302–303.
Buide, M. L. (2004). Intra-inflorescence Variation in Floral Traits and Reproductive Success of the Hermafrodite Silene acutifolia. Ann. Bot. 94, 441–448. doi: 10.1093/aob/mch164
Butcher, D., and Gouda, E. (2017). The New Bromeliad Taxon List, version 4. Available online at: https://bromeliad.nl/taxonlist/ (accessed on May 17, 2022).
Caruso, C. M., Remington, D. L. D., and Ostergren, K. E. (2005). Variation in resource limitation of plant reproduction influences natural selection on floral traits of Asclepias syriaca. Oecologia 146, 68–76. doi: 10.1007/s00442-005-0183-4
Cascante-Marín, A., De Jong, M., Borg, E. D., Oostermeijer, J. G. B., Wolf, J. H. D., and Den Nijs, J. C. M. (2006). Reproductive strategies and colonizing ability of two sympatric epiphytic bromeliads in a tropical premontane area. Int. J. Plant Sci. 167, 1187–1195.
Cascante-Marín, A., Oostermeijer, G., Wolf, J., and Fuchs, E. J. (2014). Genetic Diversity and Spatial Genetic Structure of an Epiphytic Bromeliad in Costa Rican Montane Secondary Forest Patches. Biotropica 46, 425–432.
Colas, B., Olivieri, I., and Riba, M. (2001). Spatio-temporal variation of reproductive success and conservation of the narrow-endemic Centaurea corymbosa (Asteraceae). Biol. Conserv. 99, 375–386.
Doyle, J. J., and Dickson, E. E. (1987). Preservation of Plant Samples for DNA Restriction Endonuclease Analysis. Taxon 36, 715–722.
Dziedzioch, C., Steven, S. A. D., and Gottsberger, G. (2003). The hummingbird plant community of a tropical montane rain forest in Southern Ecuador. Plant Biol. 5, 331–337.
Eckert, C. G., Kalisz, S., Geber, M. A., Sargent, R., Elle, E., Cheptou, P.-O., et al. (2010). Plant mating systems in a changing world. Trends Ecol. Evol. 25, 35–43. doi: 10.1016/j.tree.2009.06.013
Eckert, C. G., Ozimec, B., Herlihy, C. R., Griffin, C. A., and Routley, M. B. (2009). Floral morphology mediates temporal variation in the mating system of a self-compatible plant. Ecology 90, 1540–1548. doi: 10.1890/08-1063.1
Eckert, C. G., Samis, K. E., and Lougheed, S. C. (2008). Genetic variation across species’ geographical ranges: the central-marginal hypothesis and beyond. Mol. Ecol. 17, 1170–1188. doi: 10.1111/j.1365-294X.2007.03659.x
Excoffier, L., Smouse, P. E., and Quattro, J. M. (1992). Analysis of molecular variance inferred from metric distances among DNA haplotypes: application to human mitochondrial DNA restriction data. Genetics 131, 479–491. doi: 10.1093/genetics/131.2.479
Fleming, T. H., and Muchhala, N. (2008). Nectar-feeding bird and bat niches in two worlds: pantropical comparisons of vertebrate pollination systems. J. Biogeogr. 35, 764–780.
Frankham, R., Bradshaw, C. J. A., and Brook, B. W. (2014). Genetics in conservation management: revised recommendations for the 50/500 rules, Red List criteria and population viability analyses. Biol. Conserv. 170, 56–63.
Givnish, T. J., Barfuss, M. H. J., Ee, B. V., Riina, R., Schulte, K., Horres, R., et al. (2011). Phylogeny, adaptive radiation, and historical biogeography in Bromeliaceae: insights from an eight-locus plastid phylogeny. Am. J. Bot. 98, 872–895. doi: 10.3732/ajb.1000059
Givnish, T. J., Barfuss, M. H. J., Ee, B. V., Riina, R., Schulte, K., Horres, R., et al. (2014). Adaptive radiation, correlated and contingent evolution, and net species diversification in Bromeliaceae. Mol. Phylogenet. Evol. 71, 55–78. doi: 10.1016/j.ympev.2013.10.010
Godoy, F. M. R., Lenzi, M. F., Ferreira, B. H. S., Vicente-Silva, L., Zanella, C. M., and Paggi, G. M. (2018). High genetic diversity and moderate genetic structure in the self-incompatible, clonal Bromelia hieronymi (Bromeliaceae). Bot. J. Linn. Soc. 187, 672–688.
Goetze, M., Palma-Silva, C., Zanella, C. M., and Bered, F. (2016). East-to-west genetic structure in populations of Aechmea calyculata (Bromeliaceae) from the southern Atlantic rainforest of Brazil. Bot. J. Linn. Soc. 181, 477–490.
Gomes, A. C., Ferreira, B. H. S., Souza, C. S., Arakaki, L. M. M., Aoki, C., Paggi, G. M., et al. (2019). Adaptive response of extreme epiphyte Tillandsia species (Bromeliaceae) is demonstrated by different sexual reproduction strategies in the Brazilian Chaco. Bot. J. Linn. Soc. 192, 840–854.
Gonçalves-Oliveira, R. C., Wöhrmann, T., Benko-Iseppon, A. M., Krapp, F., Alves, M., Wanderley, M. G. L., et al. (2017). Population genetic structure of the rock outcrop species Encholirium spectabile (Bromeliaceae): the role of pollination vs. seed dispersal and evolutionary implications. Am. J. Bot. 104, 868–878. doi: 10.3732/ajb.1600410
Hamrick, J. L., and Godt, M. J. W. (1996). Effects of life history traits on genetic diversity in plant species. Philos. Trans. R. Soc. Lond. B Biol. Sci. 351, 1291–1298.
Hmeljevski, K. V., Freitas, L., Domingues, R., Pereira, A. R., Cancio, A. S., Andrade, A. C. S., et al. (2014). Conservation assessment of an extremely restricted bromeliad highlights the need for population-based conservation on granitic inselbergs of the Brazilian Atlantic Forest. Flora 209, 250–259.
Hmeljevski, K. V., Reis, A., Montagna, T., and Reis, M. S. (2011). Genetic diversity, genetic drift and mixed mating system in small subpopulations of Dyckia ibiramensis, a rare endemic bromeliad from Southern Brazil. Conserv. Genet. 12, 761–769.
Hoban, S., Campbell, C. D., Silva, J. M., Ekblom, R., Funk, W. C., Garner, B. A., et al. (2021). Genetic diversity is considered important but interpreted narrowly in country reports to the Convention on Biological Diversity: current actions and indicators are insufficient. Biol. Conserv. 261:109233. doi: 10.1016/j.biocon.2021.109233
Huang, S.-Q., and Guo, Y.-H. (2002). Variation of pollination and resource limitation in a low seed-set tree, Liriodendron chinense (Magnoliaceae). Bot. J. Linn. Soc. 140, 31–38.
Jacquemyn, H., Meester, L., Jongejans, E., and Honnay, O. (2012). Evolutionary changes in plant reproductive traits following habitat fragmentation and their consequences for population fitness. J. Ecol. 100, 76–87.
Johnson, S. D., Neal, P. R., Peter, C. I., and Edwards, T. J. (2004). Fruiting failure and limited recruitment in remnant populations of the hawkmoth-pollinated tree Oxyanthus pyriformis subsp. pyriformis (Rubiaceae). Biol. Conserv. 120, 31–39.
Johnston, M. O., Porcher, E., Cheptou, P.-O., Eckert, C. G., Elle, E., Geber, M. A., et al. (2009). Correlations among Fertility Components Can Maintain Mixed Mating in Plants. Am. Nat. 173, 1–11. doi: 10.1086/593705
Kamke, R., Schmid, S., Zillikens, A., Lopes, B. C., and Steiner, J. (2011). The importance of bees as pollinators in the short corolla bromeliad Aechmea caudata in southern Brazil. Flora 206, 749–756.
Keller, L. F., and Waller, D. M. (2002). Inbreeding effects in wild populations. Trends Ecol. Evol. 17, 230–241.
Kessler, M., and Krömer, T. (2000). Patterns and ecological correlates of pollination modes among Bromeliad communities of Andean forests in Bolivia. Plant Biol. 2, 659–669.
Knight, T. M., Steets, J. A., Vamosi, J. C., Mazer, S. J., Burd, M., Campbell, D. R., et al. (2005). Pollen Limitation of Plant Reproduction: pattern and Process. Annu. Rev. Ecol. Evol. Syst. 36, 467–497.
Kramer, A. T., and Havens, K. (2009). Plant conservation genetics in a changing world. Trends Plant Sci. 14, 599–607. doi: 10.1016/j.tplants.2009.08.005
Krömer, T., and Kessler, M. (2006). Distribution and Flowering Ecology of Bromeliads along Two Climatically Contrasting Elevational Transects in the Bolivian Andes. Biotropica 38, 183–195.
Krömer, T., Kessler, M., Lohaus, G., and Schmidt-Lebuhn, A. N. (2008). Nectar sugar composition and concentration in relation to pollination syndromes in Bromeliaceae. Plant Biol. 10, 502–511. doi: 10.1111/j.1438-8677.2008.00058.x
Leimu, R., Mutikainen, P., Koricheva, J., and Fischer, M. (2006). How general are positive relationships between plant population size, fitness and genetic variation? J. Ecol. 94, 942–952.
Lenzi, M., and Paggi, G. M. (2020). Reproductive biology of Dyckia excelsa Leme (Bromeliaceae): a xerophyte species from ironstone outcrops in central-western Brazil. Plant Species Biol. 35, 97–108.
Lienert, J. (2004). Habitat fragmentation effects on fitness of plant populations – a review. J. Nat. Conserv. 12, 53–72.
Lloyd, D. G. (1992). Self- and cross-fertilization in Plants. II. The selection of self-fertilization. Int. J. Plant Sci. 153, 370–380.
Lloyd, D. G., and Schoen, D. J. (1992). Self- and cross-fertilization in Plants. I. Functional dimensions. Int. J. Plant Sci. 153, 358–369.
Loveless, M. D., and Hamrick, J. L. (1984). Ecological determinants of genetic structure in plant populations. Annu. Rev. Ecol. Evol. Syst. 15, 65–95.
Marques, J. S., Tagliati, M. C., and Faria, A. P. G. (2015). Diurnal versus nocturnal pollination success in Billbergia horrida Regel (Bromeliaceae) and the first record of chiropterophily for the genus. An. Acad. Bras. Ciênc. 87, 835–842. doi: 10.590/0001-3765201520140340
Moeller, D. A., Runquist, R. D. B., Moe, A. M., Geber, M. A., Goodwillie, C., Cheptou, P.-O., et al. (2017). Global biogeography of mating system variation in seed plants. Ecol. Lett. 20, 375–384. doi: 10.1111/ele.12738
Murren, C. J. (2002). Effects of habitat fragmentation on pollination: pollinators, pollinia viability and reproductive success. J. Ecol. 90, 100–107.
Nunes, C. E. P., Briet, J., Galetto, L., Sazima, M., and Amorim, F. W. (2018). Nectar ecology of the endemic epiphytic hummingbird-pollinated bromeliad Vriesea altodaserrae: secretion dynamics and pollinator visitation pattern. Acta Bot. Bras. 32, 479–486.
Oakley, C. G., and Winn, A. A. (2012). Effects of population size and isolation on heterosis, mean fitness, and inbreeding depression in a perennial plant. New Phytol. 196, 261–270. doi: 10.1111/j.1469-8137.2012.04240.x
Ohara, M., Tomimatsu, H., Takada, T., and Kawano, S. (2006). Importance of life-history studies for conservation of fragmented populations: A case study of the understory herb, Trillium camschatcense. Plant Species Biol. 21, 1–12.
Ottewell, K. M., Bickerton, D. C., Byrne, M., and Lowe, A. J. (2016). Bridging the gap: a genetic assessment framework for population-level threatened plant conservation prioritization and decision-making. Divers. Distrib. 22, 174–188.
Paggi, G. M., Palma-Silva, C., and Bered, F. (2021). Pollination by hummingbirds of Vriesea gigantea Gaudich. (Bromeliaceae) populations in Southern Brazil. Rodriguésia 72:e01392019. doi: 10.1590/2175-7860202172056
Paggi, G. M., Palma-Silva, C., Bodanese-Zanettini, M. H., Lexer, C., and Bered, F. (2015). Limited pollen flow and high selfing rates toward geographic range limit in an Atlantic Forest bromeliad. Flora 211, 1–10.
Paggi, G. M., Palma-Silva, C., Silveira, L. C. T., Kaltchuk-Santos, E., Bodanese-Zanettini, M. H., and Bered, F. (2007). Fertility of Vriesea gigantea Gaud. (Bromeliaceae), in Southern Brazil. Am. J. Bot. 94, 683–689. doi: 10.3732/ajb.94.4.683
Paggi, G. M., Sampaio, J. A. T., Bruxel, M., Zanella, C. M., Goetze, M., Büttow, M. V., et al. (2010). Seed dispersal and population structure in Vriesea gigantea, a bromeliad from the Brazilian Atlantic Rainforest. Bot. J. Linn. Soc. 164, 317–325.
Paggi, G. M., Silveira, L. C. T., Zanella, C. M., Bruxel, M., Bered, F., Kaltchuk-Santos, E., et al. (2013). Reproductive system and fitness of Vriesea friburgensis, a self-sterile bromeliad species. Plant Species Biol. 28, 169–176.
Palma-Silva, C., Cavallari, M. M., Barbará, T., Lexer, C., Gimenes, M. A., Bered, F., et al. (2007). A set of polymorphic microsatellite loci for Vriesea gigantea and Alcantarea imperialis (Bromeliaceae) and cross-amplification in other bromeliad species. Mol. Ecol. Notes 7, 654–657.
Palma-Silva, C., Lexer, C., Paggi, G. M., Barbará, T., Bered, F., and Bodanese-Zanettini, M. H. (2009). Range-wide patterns of nuclear and chloroplast DNA diversity in Vriesea gigantea (Bromeliaceae), a Neotropical forest species. Heredity 103, 503–512. doi: 10.1038/hdy.2009.116
Palma-Silva, C., Paggi, G. M., Felicetti, R. A., Ferraz, R. S., Kaltchuk-Santos, E., Bered, F., et al. (2008). Meiotic behavior and pollen viability of wild populations of the neotropical species Vriesea gigantea (Bromeliaceae). Plant Species Biol. 23, 217–221.
Pannell, J. R., Auld, J. R., Brandvain, Y., Burd, M., Busch, J. W., Cheptou, P.-O., et al. (2015). The scope of Baker’s law. New Phytol. 208, 656–667. doi: 10.1111/nph.13539
Pansarin, E. R., and de Pedro, S. E. M. (2016). Reproductive biology of a hummingbird-pollinated Billbergia: light influence on pollinator behaviour and specificity in a Brazilian semi-deciduous forest. Plant Biol. 18, 920–927. doi: 10.1111/plb.12496
Porcher, E., and Lande, R. (2005). The evolution of self-fertilization and inbreeding depression under pollen discounting and pollen limitation. J. Evol. Biol. 18, 497–508. doi: 10.1111/j.1420-9101.2005.00905.x
Ratto, F., Simmons, B. I., Spake, R., Zamora-Gutierrez, V., MacDonald, M. A., Merriman, J. C., et al. (2018). Global importance of vertebrate pollinators for plant reproductive success: a meta-analysis. Front. Ecol. Environ. 16:82–90. doi: 10.1002/fee.1763
Reed, D. H., and Frankham, R. (2003). Correlation between Fitness and Genetic Diversity. Conserv. Biol. 17, 230–237.
Reitz, R. (1983). Bromeliáceas e a malária - bromélia endêmica. Itajaí, Santa Catarina: Herbário Barbosa Rodrigues.
Ritland, K. (1990). A series of FORTRAN computer programs for estimating plant mating systems. J. Hered. 81, 235–237.
Ritland, K. (2002). Extensions of models for the estimation of mating systems using n independent loci. Heredity 88, 221–228. doi: 10.1038/sj.hdy.6800029
Ritland, K., and Jain, S. (1981). A model for the estimation of outcrossing rate and gene frequencies using n independent loci. Heredity 47, 35–52.
Robledo-Arnuncio, J. J., Austerlitz, F., and Smouse, P. E. (2007). POLDISP: a software package for indirect estimation of contemporary pollen dispersal. Mol. Ecol. Notes 7, 763–766.
Rodger, J. G., Bennett, J. M., Razanajatovo, M., Knight, T. M., van Kleunen, M., Ashman, T.-L., et al. (2021). Widespread vulnerability of flowering plant seed production to pollinator declines. Sci. Adv. 7:eabd3524. doi: 10.1126/sciadv.abd3524
Ruas, R. B., Paggi, G. M., Aguiar-Melo, C., Hirsch, L. D., and Bered, F. (2020). Strong genetic structure in Dyckia excelsa (Bromeliaceae), an endangered species found on ironstone outcrops in Pantanal. Brazil. Bot. J. Linn. Soc. 192, 691–705.
Sampaio, J. A. T., Paggi, G. M., Zanella, C. M., Bruxel, M., Palma-Silva, C., Goetze, M., et al. (2012). Inbreeding depression in Vriesea gigantea, a perennial bromeliad from Southern Brazil. Bot. J. Linn. Soc. 169, 312–319.
Sazima, M., Buzato, S., and Sazima, I. (1999). Bat-pollinated Flower Assemblages and Bat Visitors at Two Atlantic Forest Sites in Brazil. Ann. Bot. 83, 705–712.
Schemeske, D., and Lande, R. (1985). The Evolution of Self-Fertilization and Inbreeding Depression in Plants. 2. Empirical Observations. Evolution 39, 41–52.
Schmid, S., Schmid, V. S., Zillikens, A., Harter-Marques, B., and Steiner, J. (2011a). Bimodal pollination system of the bromeliad Aechmea nudicaulis involving hummingbirds and bees. Plant Biol. 13, 41–50. doi: 10.1111/j.1438-8677.2010.00348.x
Schmid, S., Schmid, V. S., Zillikens, A., and Steiner, J. (2011b). Diversity of flower visitors and their role for pollination in the ornithophilous bromeliad Vriesea friburgensis in two different habitats in southern Brazil. Ecotropica 17, 91–102.
Schoen, D. J., and Lloyd, D. G. (1992). Self- and cross-fertilization in Plants. III. Methods for studying modes and functional aspects of self-fertilization. Int. J. Plant Sci. 153, 381–393.
Segelbacher, G., Bosse, M., Burger, P., Galbusera, P., Godoy, J. A., Helsen, P., et al. (2021). New developments in the field of genomic technologies and their relevance to conservation management. Conserv. Genet. 23, 217–242. doi: 10.1007/s10592-021-01415-5
Smouse, P. E., Dyer, R. J., Westfall, R. D., and Sork, V. L. (2001). Two-Generation analysis of pollen flow across a landscape. I. Male gamete heterogeneity among females. Evolution 55, 260–271. doi: 10.1111/j.0014-3820.2001.tb01291.x
Stockwell, C. A., Hendry, A. P., and Kinnison, M. T. (2003). Contemporary evolution meets conservation biology. Trends Ecol. Evol. 18, 94–101.
Sun, M., and Ritland, K. (1998). Mating system of yellow starthistle (Centaurea solstitialis), a successful colonizer in North America. Heredity 80, 225–232.
Tian, H., Harder, L. D., Wang, A.-Y., Zhang, D.-Y., and Liao, W.-J. (2022). Habitat effects on reproductive phenotype, pollinator behavior, fecundity and mating outcomes of a bumble-bee pollinated herb. Am. J. Bot. 109, 470–485. doi: 10.1002/ajb2.1826
Vicente-Silva, L., Godoy, F. M. R., Faggioni, G. P., Lorenz, A. P., and Paggi, G. M. (2021). Patchily distributed but not necessarily isolated populations of Deuterocohnia meziana: a threatened bromeliad from rock outcrops. Bot. J. Linn. Soc. 199, 312–330. doi: 10.1093/botlinnean/boab087
Victoriano-Romero, E., Valencia-Díaz, S., Toledo-Hernández, V. H., and Flores-Palacios, A. (2017). Dispersal limitation of Tillandsia species correlates with rain and host structure in a central Mexican tropical dry forest. PLoS One 12:e0171614. doi: 10.1371/journal.pone.0171614
Winkler, M., Hülberb, K., and Hietza, P. (2007). Population dynamics of epiphytic bromeliads: life strategies and the role of host branches. Basic Appl. Ecol. 8, 183–196.
Wolowski, M., Saad, C. F., Ashman, T.-L., and Freitas, L. (2013). Predominance of self-compatibility in hummingbird-pollinated plants in the Neotropics. Naturwissenschaften 100, 69–79. doi: 10.1007/s00114-012-0995-0
Zanella, C. M., Janke, A., Palma-Silva, C., Kaltchuk-Santos, E., Pinheiro, F. G., Paggi, G. M., et al. (2012). Genetics, Evolution, and Conservation of Bromeliaceae. Genet. Mol. Biol. 35, 1020–1026. doi: 10.1590/s1415-47572012000600017
Keywords: Atlantic Forest, contemporary gene flow, microsatellite loci, selfing rate, Southern Brazil, Tillandsioideae
Citation: Paggi GM, Palma-Silva C, Zanella CM, Goetze M, Büttow MV, Lexer C and Bered F (2022) Spatiotemporal Variation on Fertility, Mating System, and Gene Flow in Vriesea gigantea (Bromeliaceae), an Atlantic Forest Species. Front. For. Glob. Change 5:893548. doi: 10.3389/ffgc.2022.893548
Received: 10 March 2022; Accepted: 16 May 2022;
Published: 16 June 2022.
Edited by:
Fernanda Amato Gaiotto, Universidade Estadual de Santa Cruz, BrazilReviewed by:
Jose Climent, Instituto Nacional de Investigación y Tecnología Agroalimentaria (INIA), SpainThorsten Krömer, Universidad Veracruzana, Mexico
Copyright © 2022 Paggi, Palma-Silva, Zanella, Goetze, Büttow, Lexer and Bered. This is an open-access article distributed under the terms of the Creative Commons Attribution License (CC BY). The use, distribution or reproduction in other forums is permitted, provided the original author(s) and the copyright owner(s) are credited and that the original publication in this journal is cited, in accordance with accepted academic practice. No use, distribution or reproduction is permitted which does not comply with these terms.
*Correspondence: Gecele Matos Paggi, gecele.paggi@ufms.br
†Deceased