- 1African Sustainable Agriculture Research Institute (ASARI), Mohammed VI Polytechnic University (UM6P), Laâyoune, Morocco
- 2AgroBioSciences Department, Mohammed VI Polytechnic University (UM6P), Benguerir, Morocco
- 3Department of Biology, Bahir Dar University, Bahir Dar, Ethiopia
- 4Laboratoire Biotechnologies Microbiennes, Agrosciences et Environnement (BioMagE), Unité de Recherche Labellisée CNRST N°4, Faculty of Science Semlalia, Cadi Ayyad University, Marrakesh, Morocco
- 5Institut National de la Recherche Agronomique (INRA), Rabat, Morocco
Eichhornia crassipes (Mart.) Solms, commonly known as water hyacinth, is one of the world’s most invasive aquatic plants of the Pontederiaceae family occurring in tropical and subtropical regions of the world. Although, E. crassipes causes significant ecological and socioeconomic issues such as a high loss in water resources, it has multipurpose applications since it is famous for many industrial applications such as bioenergy, biofertilizer production, wastewater treatment (absorption of heavy metals), and animal feed. Furthermore, E. crassipes is rich in diverse bioactive secondary metabolites including sterols, alkaloids, phenolics, flavonoids, tannins, and saponins. These secondary metabolites are well known for a wide array of therapeutic properties. The findings of this review suggest that extracts and some isolated compounds from E. crassipes possess some pharmacological activities including anticancer, antioxidant, anti-inflammatory, antimicrobial, skin whitening, neuroprotective, and hepatoprotective activities, among other biological activities such as allelopathic, larvicidal, and insecticidal activities. The present review comprehensively summarizes the chemical composition of E. crassipes, reported to date, along with its traditional uses and pharmacological and biological activities.
Introduction
Eichhornia crassipes (Mart.), commonly known as water hyacinth, is a monocotyledonous free-floating aquatic plant belonging to the family Pontederiaceae. The plant is native to Brazil and the Amazon, but it has been naturalized in tropical and subtropical regions. It has also been reported in several parts of Africa, including Egypt, Sudan, Kenya, Ethiopia, Nigeria, Zimbabwe, Zambia, and South Africa (Dersseh et al., 2019). The plant is characterized by its high growth, rapid and extensive spread, and strong tolerance to pH and nutrient variations as well as temperature conditions. Hence, it has been recognized by the International Union for Conservation of Nature as one of the 100 most aggressive invasive species and identified as one of the 10 severest weed plants in the world (Téllez et al., 2008; Zhang et al., 2010; Patel, 2012). However, E. crassipes possesses many potential benefits but with financial and environmental fallout (Yan et al., 2017; Su et al., 2018). It has been used as phytoremediation agent for wastewater treatments because of its ability to absorb heavy metals and grow in polluted water (Mishra and Maiti, 2017; Mustafa and Hayder, 2021). It has also been considered as a potential source of bioenergy (Carreño Sayago and Rodríguez, 2018) and biofertilizers (Manyuchi et al., 2019). Traditionally, the plant is used to treat gastrointestinal disorders, such as diarrhea, intestinal worms, digestive disorders, and flatulence. In addition, the beans were harnessed for healthy spleen functioning (Sharma et al., 2020). The plant is also rich in various bioactive compounds that exhibit a wide array of pharmacological properties.
These include antioxidant (Liu et al., 2018), antimicrobial (Chang and Cheng, 2016), antitumor (Ali et al., 2009), anticancer (Aboul-Enein et al., 2014), anti-inflammatory (Jayanthi et al., 2013) as well as hepatoprotective (Kumar et al., 2014), larvicidal (Turnipseed et al., 2018), and wound healing (Lalitha and Jayanthi, 2014). Many patents have also been filed, mainly in the fields of medicinal uses of the plant and its product formulations.
The current review comprehensively assesses the state of the art concerning the phytochemical composition, therapeutic uses, and pharmaceutical applications of E. crassipes (Mart.) along with patents reported on the plant.
Methodology of Research
A literature-based search was conducted to provide an overview of the phytochemistry, value-added products, and pharmacological activities of E. crassipes, using accessible online databases such as PubMed, Scopus, Web of Science, and Google Scholar. The literature survey was performed using different keywords including “Eichhornia crassipes” or “water hyacinth” and chemical constituents, or value-added products, or antioxidant, or anti-inflammatory, or antimicrobial or hepatoprotective or wound healing, which resulted in the gathering of much literature. An extensive number of studies published in research articles, review articles, book chapters, and books were collected. From 2,835 identified studies, a total of 150 studies, which met the inclusion criteria, were preserved in this survey. The outline for literature search and management is presented in Figure 1.
Botanical Description
The Pontederiaceae family possesses nine genera, including Eichhornia. The latter is composed of eight species of aquatic plants, among them is Eichhornia crassipes (Mart.) Solms: synonym of Pontederia crassipes (Mart.). The mature plant has roots, leaves, stolon, inflorescences, and fruit clusters (Parsons and Cuthbertson, 2001) (Figure 2). The root morphology is highly plastic and fibrous, having one single main root with many laterals, forming a huge root system. Because each lateral root has a root tip, E. crassipes may exploit nutrients in a low-nutrient water body, which makes the lateral roots longer and denser at low phosphorus concentrations.
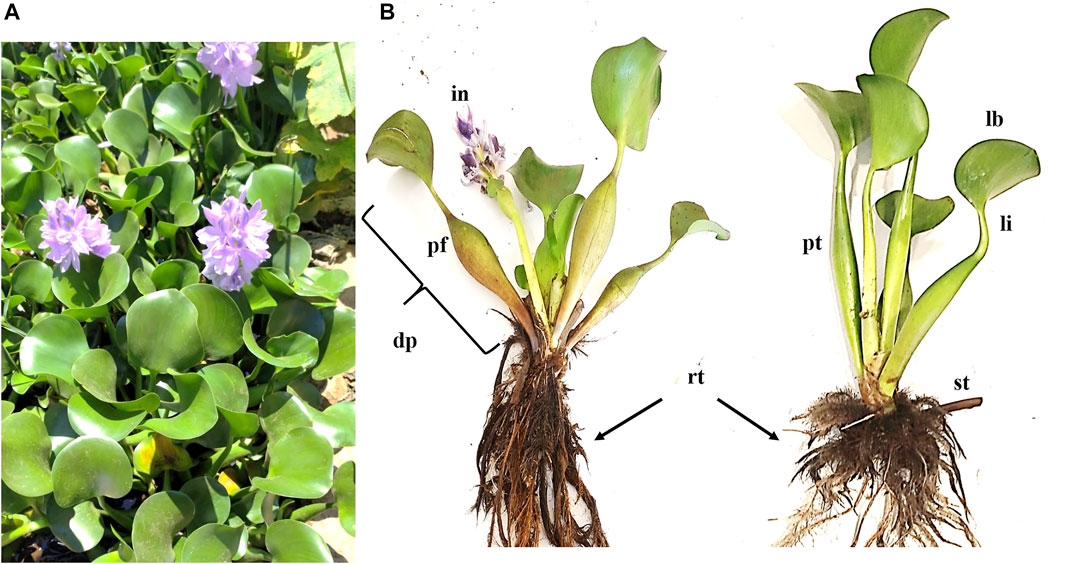
FIGURE 2. (A) Eichhornia crassipes (Mart.) from Lake Tana, Ethiopia. (B) Morphology of E. crassipes. rt: root; st: stolon; pt: petiole; lb: leaf blade; li: leaf isthmus; dp: daughter plant; in: inflorescence; pf: peduncle of flower spike.
E. crassipes petioles are both erect and horizontal as stolon. There are two types of leaves, thin and round. The thin ones stand erect while the round ones possess a slightly undulating edge. In addition, the two types of leaves are soft, glossy, and glabrous. The leaves possess semi-parallel veins following their curvature (Parsons and Cuthbertson, 2001). The plant possesses beautiful violet flowers with six petals that may be found throughout the year under favorable conditions. However, the intensity of flowering may differ over the four seasons. The fruit contains 300 seeds in a slim three-celled capsule which measures 1–1.5 mm long with many longitudinal ribs. In regions with temperatures around 25°C, the seeds can remain inactive for up to 20 years and then germinate with water. Generally, temperatures between 20 and 35°C enhance germination while temperatures around 35°C enhance rapid growth (Parsons and Cuthbertson, 2001; Malik, 2007).
Phytochemistry
The phytochemical composition of E. crassipes has been extensively explored, revealing diverse secondary metabolites, among them polyphenols (9.73%), flavonoids (10.49%), fatty acids (10.1%), alkaloids (7.4%), sterols (6.17%), and other compounds (19.13%) as shown in Figure 3. Several primary metabolites were annotated from the different parts of the plant, which include heteropolysaccharides such as L-galactose, L-arabinose, and D-xylose (Anjaneyalu et al., 1983), as well as hemicellulose, cellulose, glycolipids, and triacylglycerols (Balasubramanian et al., 2012). Phosphatidylethanolamine, phosphatidylcholine, and phosphatidylglycerol are the main phospholipids identified in the flowers, leaves, stalks, and roots (Lalitha and Jayanthi, 2012). The leaves contain several amino acids and are mainly rich in leucine, asparagine, and glutamine (Virabalin et al., 1993). Two fractions of peptides have also been identified from the leaves as Leu-Phe and Phe-Phe-Glu (Zhang et al., 2018).
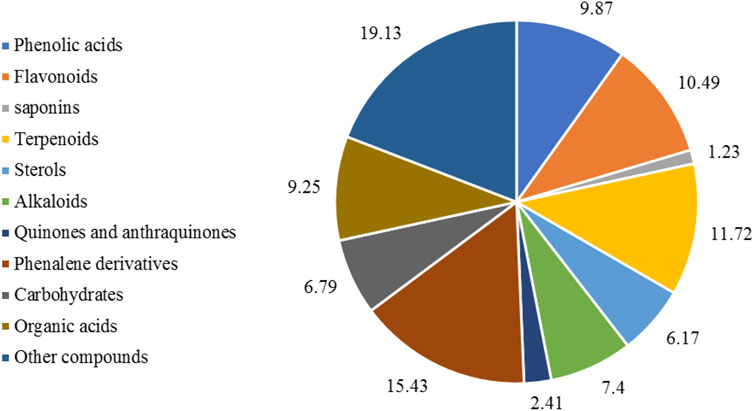
FIGURE 3. Major classes and subclasses of compounds isolated from E. crassipes based on the total number of phytochemicals. The keywords used on the Web of Science were “E. crassipes,” OR “water hyacinth” “phytocompounds,” “secondary metabolites,” “phenolic,” “flavonoids,” “saponins,” “sterols,” “terpenoids,” “carbohydrates,” “quinones,” “tannins,” “organic acids,” and “other compounds.”
Phenolic Compounds
Many studies have identified and quantified phenolic compounds in E. crassipes (Surendraraj et al., 2013; Tyagi and Agarwal, 2017b). Simple phenols were identified in different extracts from different parts of E. crassipes collected from India. They are represented by pyrogallol (1), 4-methylresorcinol (2), catechol (3), 2-methylresorcinol (4), and resorcinol (5). Moreover, many phenolic acids were detected in different types of extracts of the leaves, petioles, and flowers of E. crassipes. They are represented by p-hydroxybenzoic (6), gentisic (7), chlorogenic (8), caffeic (9), p-coumaric (10), ferulic (11), vanillic (12), syringic (13), gallic (14), protocatechuic (15), and salicylic acids (16) (Lata and Dubey, 2010; Surendraraj et al., 2013). The ethanolic extract of flowers contained higher levels of gentisic, protocatechuic acids, and p-hydroxybenzoic acid than that of the petioles and leaves (Surendraraj et al., 2013). The chemical structures of phenolic compounds are illustrated in Supplementary Figure S1.
Flavonoids
E. crassipes extracts are rich in flavonoids and their glycosides (Lata and Dubey, 2010; Jayanthi et al., 2011). The aqueous and petroleum extracts of the rhizome and shoot were characterized by the presence of gossypetin (17), tricin (18), azaleatin (19) chrysoeriol (20), luteolin (21), and apigenin (22). In addition, orientin (23), kaempferol (24), quercetin (25), and isovitexin (26) were also identified from the roots and shoots (Nyananyo et al., 2007; Lata and Veenapani, 2010; Jayanthi et al., 2011). Naringenin (27), kaempferol (24), myricetin (28), and rutin (29) were reported in the leaves and petiole (Chantiratikul et al., 2009). Quercetin 7-methyl ether (30) was recently isolated from the ethanol extract of the plant (Elvira et al., 2018). An acylated delphinidin glycoside represented by 6‴-O-{delphinidin 3-O-[6‴-O-(β-d-glucopyranosyl)]} {6‴-O-[apigenin 7-O-(β-d-glucopyranosyl)]} malonate (31) was isolated from the flowers and was not detected in any other parts of the plant (Toki et al., 1994) (Supplementary Figure S2). Anthocyanins, a subgroup of flavonoids, have been detected in the ethanol, acetone, and aqueous extracts of the shoots and leaves parts of E. crassipes collected from India (Jayanthi et al., 2011).
Saponins
Many studies have confirmed the presence of saponins in various extracts of different parts of E. crassipes (Baral and Vaidya, 2011; Jayanthi et al., 2011; Hamid et al., 2013; Anusiya et al., 2020). Saponins were detected in the aqueous extracts from samples collected from the Phewa Lake in Nepal (Baral and Vaidya, 2011). By contrast, aqueous extracts of the plant from Dijla River, Baghdad, showed the absence of saponins (Hamid et al., 2013). Moreover, the phytochemical screening of hexane, chloroform, and ethanol extracts revealed the presence of saponins from samples collected from Nepal (Baral and Vaidya, 2011; Baral et al., 2012; Lalitha and Jayanthi, 2012). For instance, two steroidal saponins, namely spirostane (32) and cholestane (33) were isolated from E. crassipes. The first was found in the acetone extract of the roots and the second in the cyclohexane leaf extract of E. crassipes (Fileto-Pérez et al., 2015) as shown in Supplementary Figure S3. These compounds characterized the plant collected from India and were not detected elsewhere.
Terpenoids
Phytol (34) was identified by GC-MS in the ethanol extract from the whole plant collected from India (Muthunarayanan et al., 2011; Tyagi and Agarwal, 2017a). This compound is considered a major bioactive compound present in the leaves of the plants collected from India (Tyagi and Agarwal, 2017a; Kumar et al., 2018a). Squalene (35), a hypocholesterolemic terpenoid, was identified in the non-polar and polar extracts of the leaves and stems of E. crassipes, from Mexico (Supplementary Figure S4) (Fileto-Pérez et al., 2015). This compound has been only identified in the Mexican plant. GC-MS studies conducted by Lenora et al. (2016) have reported the presence camarolide (36), a pentacyclic triterpenoid, in the methanol extract of the aerial parts of the plant.
Fatty Acids
The GC-MS analysis of the leaves of E. crassipes revealed the presence of many fatty acids represented by linolenic acid, ethyl ester (37) (26.26%), palmitic acid ethyl ester (38) (12.09%), α-glyceryl linolenate (39) (1.35%), E-11-hexadecenoic acid, ethyl ester (40) (1.04%), and stearic acid, ethyl ester (41) (0.98%). The GC-MS of the petiole part revealed the presence of hexadecanoic acid, ethyl ester, synonym of palmitic acid, ethyl ester (37) (23.7%), 9,12,15-octadecatrienoic acid, ethyl ester, (Z,Z,Z) (42) (5.50%), and n-hexadecanoic acid (43) (3.82%) (Tyagi and Agarwal, 2017b). The latter was also identified in the shoot extracts (Anusiya et al., 2020). Other fatty acids were identified in different types of extracts from the leaves, stems, and roots. These include linolenic acid (44), caprylic acid (45), lauric acid (46), myristic acid (47), oleic acid (48), vaccenic acid (49), tetracosanoic acid (50), and 10,12-octadecadienoic acid (51), and cis-vaccenic acid (52) (Fileto-Pérez et al., 2015; Adelodun et al., 2020) (Supplementary Figure S5).
Sterols
Phytosterols are steroidal molecules with a similar structure to cholesterol found in many vegetables (Wasowicz and Rudzinska, 2011). Sterols represent 19–23% wt. of the extracts of E. crassipes. 6α-Hydroxystigmata-4,22-dien-3-one (53), 4α-methyl-5α-ergosta-7,24(28)-diene-3β,4β-diol (54), 4α-methy1-5α-ergosta-8,14,24(28)-triene-3β,4β-diol (55), and 4α-methyl-5α-ergosta-8-24(28)-triene-3β,4β-diol (56) were isolated from the ethyl acetate extract of the plant (DellaGreca et al., 1991). β-campesterol (57), methylcholesterol (58), β-sitosterol (59), and sitosterol (60) were detected in the stalk and leaf extracts. The stalk parts showed the maximum content of stigmasterol (61) (Goswami et al., 1983; Silva et al., 2015; Singh et al., 2015; Martins et al., 2016; Tyagi and Agarwal, 2017a). Stigmasterol is considered the most common and major phytosterol identified in different parts of E. crassipes (Tyagi and Agarwal, 2017a; Kumar et al., 2018a). Furthermore, β-stigmasterol (62) was found in hexane, acetone, and methanolic extracts of the leaves and stems (Fileto-Pérez et al., 2015).
Recently, a novel derivative of stigmasterol named 22,23-dibromostigmasterol acetate (63) was isolated from the ethanolic extract of the shoots and amounted to 28.72% of the extract (Tyagi and Agarwal, 2017a; Anusiya et al., 2020). The structures of these chemicals are represented in the supplementary materials in Supplementary Figure S6.
Alkaloids
E. crassipes is considered a potential source of alkaloids. They represent 0.98% of the crude extract of the plant (Shanab et al., 2010). In the rhizome and shoot, tomatine (64) and cytisine (65) were found to predominate. Quinine (66), thebaine (67), and codeine (68) exist only in the shoot while nicotine (69) is found mainly in the rhizome of the Indian species (Lata and Dubey, 2010). In addition, 1H-pyrrole,1-phenyl (70) and pipradrol (71) were identified in the ethanol extract using GC-MS (Shanab et al., 2010; Shanab et al., 2011). Furthermore, 18,19-secoyohimban-19-oic acid-16,17,20,21-tetradehydro-16-(hydroxymethyl)-methyl ester (72), di amino-di-nitro-methyl dioctyl phthalate (73), and 9-(2′,2′-dimethyl-propanoilhydrazono)-2,7-bis-[2-(diethylamino)-ethoxy]fluorene (74) were isolated from leaf extracts (Supplementary Figure S7) (Aboul-Enein et al., 2014; Mtewa et al., 2018).
Quinones and Anthraquinones
The shoot extracts were reported to contain several quinones represented by aloe-emodin (75), 7-methyl-juglone (76), and rhein (77), whereas aloe-emodin (75) was found in the rhizome as well (Lata and Veenapani, 2010). Anthraquinones, on the other hand, were found in all extracts except the light petroleum fraction (Supplementary Figure S8) (Jayanthi et al., 2011; Tulika and Mala, 2015; Anusiya et al., 2020).
Phenalene and Phenylphenalene Derivatives
Permethylated phenalene derivatives were identified from E. crassipes represented by 2,6-dimethoxy-9-phenylphenalenone (78), 4,5-dimethoxy-9-phenyl-2,3-dihydrophenalen-1-ol-O-methyl ether (79), 4,9-dimethoxy-7-(4′-methoxy-phenyl)-2,3-dihydro-phenalen-1-ol-O-methyl ether (80), and 4,9-dimethoxy-7-phenyl-2,3-dihydrophenalen-1-ol-O-methyl ether (81) (DellaGreca et al., 1992). In addition, 8-phenylphenalenone compounds represented by 2-hydroxy-8-(4-hydroxyphenyl)-phenalen-1-one (82) and 2- hydroxy-8-(3,4-dihydroxyphenyl)-phenalen-1-one (83) were obtained from the acetone extract of the roots and leaves of the plant (Hölscher and Schneider, 2005).
Phenylphenalene derivatives were also identified and isolated. They were represented by 4,8,9-trimethoxy-1-phenyl-2,3-dihydro-1H-phenalene (84), 4,8,9-trimethoxy-1-(4 methoxyphenyl)-2,3-dihydro-1H-phenalene (85), 4,4″,8,8″,9,9″-hexamethoxy-1,1″-diphenyl-2,2″,3,3″-tetrahydro-7,7″-bi(1H-phenalene) (86), 4,4″,8,8″,9,9″,4′,4‴-octamethoxy-1,1″-diphenyl-2,2″,3,3″-tetrahydro-7,7″-bi(1H-phenalene) (87), 6,6″,8,8″,9,9″,4′,4‴-octamethoxy-1,1″-diphenyl-2,2″,3,3″-tetrahydro-7,7″-bi(1H-phenalene) (88), methyl 5-methoxy-2-phenyl-8[3,7,10-trimethoxy-6-phenyl-5,6-dihydro-4H-phenaleno(2,1-b)furan-9-yl]-1-naphthoate (89), 2,3-dihydro-4,8-dimethoxy-9-phenyl-1H-phenalen-1-ol (90), 2,3-dihydro-8-methoxy-9-phenyl-1H-phenalene-1,4-diol (91), 2,3-dihydro-9-(4-hydroxyphenyl)-8-methoxy-1H-phenalene-1,4-diol (92), together with 2,6-dimethoxy-9-phenyl-1H-phenalen-1-one (93), 2-hydroxy-9-(4-hydroxyphenyl)-1H-phenalen-1-one (94), and 2,3-dihydro-3,9-dihydroxy-5-methoxy-4-phenyl-1H-phenalen-1-one (95). Moreover, 5,6-dimethoxy-7-phenyl-1H-phenalen-1-one (96), 2-hydroxy-9-(4-hydroxyphenyl)-1H-phenalen-1-one (97), and methyl 3-(4-hydroxy 3-methoxyphenyl)prop-2-enoate) (98) were isolated from the ethyl acetate fraction of the whole plant (DellaGreca et al., 2008; DellaGreca et al., 2009; Wang et al., 2017). The later compounds were identified in the plant collected from Naples. Structures are represented in Supplementary Figure S9.
Carbohydrates
Sucrose (99), fructose (100), glucose (101), xylose (102), arabinose (103), and galactose (104) are the main soluble sugars present in the leaves, along with galactomannan (105) and branched (1→3)-β-D-glucan (Arifkhodzhaev and Shoyakubov, 1995). The chloroform and aqueous extracts of the shoots revealed the presence of cardiac glycosides, however, they were absent in the rhizome (Lata and Dubey, 2010). Sulfated polysaccharides were found in the whole plant, with high amounts in the roots (Dantas-Santos et al., 2012). Furthermore, cellulose xanthate was produced from the chemical treatment of E. crassipes shoot and root biomass with NaOH and CS2 (Zhou et al., 2009), which is known for its ability to adsorb heavy metals (Deng et al., 2012). Nanocrystalline cellulose was isolated from E. crassipes fibers after chemical and mechanical treatments (Asrofi et al., 2017). Xylitol (106), a pentose polyol, used in food and pharmaceutical industries, was also isolated and identified from the plant (Prakasham et al., 2009). Different studies reported the yield of xylose from E. crassipes biomass. Kalhorinia et al. (2014) reported a yield of 35 g/L of xylitol using simple and efficient acid pretreatment, while 0.25 g/L of xylitol was produced from the hemicellulosic parts of the plant by acid hydrolysis (Shankar et al., 2020). The worldwide market of xylitol is more than 700 million USD/year in the food and pharmaceutical industries and is expected to reach 1.37 USD billion by 2025. The selling price of xylitol is estimated to be 5 USD/kg (Supplementary Figure S10) (Raj and Krishnan, 2020).
Organic Acids
In total, 20 organic acids were identified in different types of extracts from the leaves, stem, and roots of the Mexican plant. These include oxalic acid (107), nonanoic acid (108), malonic acid (109), succinic acid (110), and phthalic acid (111) (Fileto-Pérez et al., 2015). While, propiolic acid (112) was identified from the ethanolic extract of the leaves as a major compound from the plant collected from India (Kumar et al., 2018a).
Furthermore, levulinic acid (113) extracted with microwave heating techniques was isolated from the dried plant with a yield of 9.43% dry weight (Lai et al., 2011). From the aerial parts, shikimic acid (114), an antiviral agent, was isolated with a yield of 0.03–3.25% w/w from 1.0 g of plant material (Bochkov et al., 2012; Cardoso et al., 2014; Lenora et al., 2016). Isoascorbic acid (115), ascorbic acid (116), and dehydroascorbic acid (117) were present in the shoot extracts, however, the latter was detected only in the rhizome (Lata and Dubey. 2010). Humic acids, which play an essential role in retaining water and texture soils were also found to be present in several parts of the plant such as the leaves, stems, and roots (Supplementary Figure S11) (Ghabbour et al., 2004).
Other Compounds
Other metabolites belonging to different classes were detected in different parts of E. crassipes.
Phenylnaphthalenedicarboxylic acids were isolated from the acid fraction of the ethyl acetate extract of E. crassipes and identified as 2-(p-methoxyphenyl)-5-methoxy-1,8-naphthalenedicarboxylic acid dimethyl ester (118), 2-phenyl-6-methoxy-1,8-naphthalenedicarboxylic acid dimethyl ester (119), 2-phenyl-1,8-naphthalenedicarboxylic acid dimethyl ester linked at C-5 to a phenalenol (120) and 2-phenyl-5-methoxy-1,8-naphthalenedicarboxylic acid dimethyl ester (121) (Greca et al., 1993).
4H-pyran-4-one, 2,3-dihydro-3,5-dihydroxy-6-methyl (122) was obtained from the ethanol extract of the leaves (Muthunarayanan et al., 2011).
Glycerol-1,9-12(ZZ)-octadecadienoic ester (123) and N-phenyl-2-naphthylamine (124) were isolated from the acetone extract of the roots (Shanyuan et al., 1992).
2,2-dimethylcyclopentanone (125), isocyanoethyl acetate (126), and propane amide (127) were separated from the acetone extract and demonstrated anti-algal activity (Jin et al., 2003). The plant collected from Mexico contained high levels of melatonin (128) and N1-acetyl-N2-formyl-5- methoxykynuramine (129), two strong free radical scavengers (Tan et al., 2007).
Moreover, 1,2-benzenedicarboxylic acid, mono-(2-ethylhexyl) ester (130), 1,2-benzenedicarboxylic acid, dioctyl ester (131), 1,2-benzenedicarboxylic acid, diisooctyl ester (132), (3-methylphenyl)-phenylmethanol (133), and 4-(diethylamino)-alpha-[4-(diethylamino) phenyl] (134) have been identified from E. crassipes extracts collected from River Nile, Egypt (Aboul-Enein et al., 2014). It is noteworthy to say that these compounds were only identified in the plant from Egypt. The GC-MS analysis of the oily fraction, extracted with n-hexane from the whole plant of E. crassipes, resulted in the identification of 18 compounds. The most abundant compounds were long-chain alcohols in addition to long-chain nitrogenous compounds like nonadecan-4-ol (135), 17-methoxydocosa-1,4,7,10-tetraene-6,9-dione (136), 9,16-dimethylnonadec-1-en-9-ol (137), tricosane-4-ol (138), 5-methoxy heneicosane (139), 1-aminooctadeca-8,10,12-trien-7-ol (140), and 6-(6-(octadeca-1,3,7,12,14,16-hexaenyl)pyridin-2-yl)hex-5-en-1-ol (141) (Hussain et al., 2015). GC-MS studies conducted by Lenora et al. (2016) have reported the presence of some chemicals in the methanol extract of the aerial parts of the plant. These include 1,8 dipropoxyanthraquinone (142), erucylamide (143), nonacosane (144), and docosane (145). GC-MS analysis of the ethanolic leaf extract led to the identification of various phytochemical compounds including 17-pentatriacontene (146) and octasiloxane (147) considered as major compounds (Kumar et al., 2018a). GC-MS analysis of the ethanolic leaves extract led to the identification of 1-monolinoleoylglycerol trimethylsilyl ether (148) (Tyagi and Agarwal, 2017a,; Kumar et al., 2018a). The compound was also identified and amounted to 30.89% of the ethanolic extracts of the roots (Kumar et al., 2018a).
Moreover, 14-heptadecenal (149), 16-heptadecenal (150), 4-methyl (phenyl)silyloxypentadecane (151), 3,6-methano-8H-1,5,7-trioxacyclopenta[1J]cyclopro [A]azulene-4,8(3H), 1,4-dioxane-2,5-dione, 3,6-dimethyl (152), 1-hexyl-2-nitrocyclohexane (153), and nonanoic acid, 5-methyl-, ethyl ester (154) were isolated from the ethanolic extract of the shoot and root parts of the plant collected from India (Supplementary Figure S12) (Anusiya et al., 2020).
Value-Added Products From E. crassipes (Mart.) Solms
The biorefinery of E. crassipes biomass revealed several enzymes and valuable products. Furfural and hydroxymethylfurfural, for instance, were produced using the nonhazardous oxidant (FeCl3) method with the highest yield of 7.9 wt% of the dry mass of the plant (Liu et al., 2018; Poomsawat et al., 2019). Moreover, due to E. crassipes availability, low price, and its high percentage of cellulose, the plant is considered a favorable source to produce fibers, superconductors, and supercapacitors (Sundari and Ramesh, 2012; Asrofi et al., 2017; Sindhu et al., 2017). The liquid tar obtained from the plant (rich in phenolic compounds) yielded 29% of carbon fiber, which makes the plant suitable for fiber production (Soenjaya et al., 2015).
In addition, different biopolymers with diverse applications along with several enzymes such as cellulase, β-glucosidase, and xylanase were obtained from the plant biomass (Table 1). The enzymes are produced from the plant residue, as carbon source, by submerged fermentation or under solid state fermentation using different microorganisms. The production of these enzymes harnessed on large for cost-effective industrial applications. Table 2 presents the different enzymes produced from E. crassipes residue.
In the same line, biopolymers are produced by various microorganisms, like Cupravidus necatar and Pseudomonas aeruginosa, combined with acid pretreatments using E. crassipes as a substrate. Radhika and Murugesan (2012) revealed that the addition of E. crassipes enzymatic hydrolizate gave 4.3 g/L of PHB.
Moreover, different chemical pretreatments along with enzymatic saccharification by Ralstonia eutropha yielded 73% of the biopolymer PHB (Saratale et al., 2020).
Meanwhile, the composites are prepared using solution impregnation and hot curving methods (Ramirez et al., 2015). According to several studies, E. crassipes has been used as a raw material to produce high-value chemicals such as furfural, enzymes, biopolymers, and composites as reviewed in Guna et al. (2017), Sindhu et al. (2017), and Ilo et al. (2020).
Pharmacological and Biological Activities
The varied ethnobotanical uses of E. crassipes have led to the ignition of various pharmacological investigations. A diverse range of in vitro and in vivo test systems has been used to evaluate the pharmacological properties of E. crassipes. Table 3 summarizes the reported pharmacological activities of E. crassipes. These include anti-microbial, antioxidant, wound healing, antitumor, and cytotoxic activities encompassing more than 50% of the studies. The activities related to larvicidal, insecticidal, and allelopathic effects accounted for 20% of the studies (Figure 4). The wide range of biological activities of E. crassipes are attributed to the presence of bioactive compounds belonging to different classes of secondary metabolites as reported earlier.
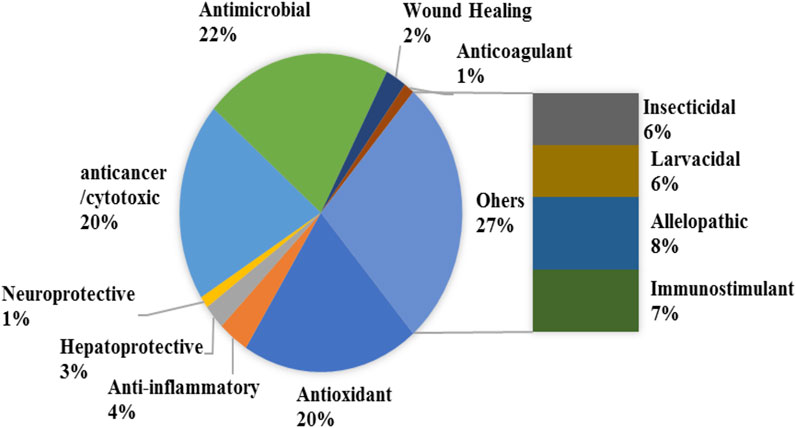
FIGURE 4. Percentage of the studied biological and pharmacological activities of E. crassipes according to the literature.
Neuropharmacological Activities
Potential behavioral neuropharmacological activities, as evidenced by the analgesic, anti-epileptic sedative, central nervous system depressant, anti-anxiety, anti-psychosis, anti-depressant, and memory-improving properties, were exerted by the ethanol extract of leaves of E. crassipes in combination with ethanol extracts of Nelumbo nucifera leaves in mice models (Farheen et al., 2015). The results showed that the ethanol extract of E. crassipes leaves significantly inhibited motor activity, demonstrated high anti-anxiety property, and decreased the exploratory behavior pattern in evasion tests. The treated mice were able to maintain their posture for over 180 s. Moreover, the same extract prolonged sleep latency and duration, improved latency period, and generated the highest inhibition of writhing test induced by acetic acid. In addition, the histopathological findings confirmed the neuronal protective properties of E. crassipes in combination with N. nucifera, where an important glial reaction was observed in the brain of the treated mice (Farheen et al., 2015). However, there are some shortcomings in the application of E. crassipes extracts as a neuroprotective agent. In this line, further studies are required to confirm the neuropharmacological activity of the plant, to clarify the correlation between the phytochemical composition and the pharmacological activity.
Anti-Inflammatory Activities
The stems and leaves of E. crassipes were used to treat swelling and wounds due to its anti-inflammatory activity associated with the phenolic content in the plant (Rorong et al., 2012). In the sample line, lemon juice plus the juice of E. crassipes leaves have been traditionally used as anti-inflammatory topical agents in the Philippines (Sharma et al., 2020).
So far, there are only few articles demonstrating the toxicity of E. crassipes. The hydroalcoholic extract of the leaves at 500 mg/kg showed no death of animals within 14 days of administration of extracts (Ali et al., 2009).
The in vivo anti-inflammatory activity of ethyl acetate, petroleum ether, and aqueous extracts of the leaves and shoot parts of the plant were studied on formaldehyde-induced paw edema. Among the studied extracts, the ethyl acetate extract showed the best anti-inflammatory activity with 67.5% of inhibition of paw edema. This anti-inflammatory activity would be related to the presence of anthocyanins and phenolic compounds (Jayanthi et al., 2011). Moreover, the investigation of the in vitro anti-inflammatory activity of the methanol extract of E. crassipes by the inhibition of albumin denaturation technique demonstrated a maximum inhibition of 79% at a concentration of 500 μg/ml (Sunitha et al., 2018). This activity could be attributed to the presence of sterols, especially stigmasterol, which has a role as an anti-inflammatory compound. Furthermore, the compound could be used as a precursor to produce other bioactive compounds for medical purposes (Paniagua-Pérez et al., 2008).
Hepatoprotective Activities
Different parts of E. crassipes have been used as a traditional herbal remedy for its beneficial effects on human diseases. In Bangladesh, the roots and flowers are used in the treatment of hepatic disorders and abdominal swelling (Rahmatullah et al., 2010).
The methanolic extract of E. crassipes demonstrated hepatoprotective activity against CCl4-induced hepatotoxicity in rats. The plant extract was effective in protecting the liver at 400 mg/kg against injury induced by CCl4 in rats manifested by a significant reduction in bilirubin (TB = 0.05 mg/Dl), serum glutamic oxaloacetic transaminase (SGOT = 293 IU/L), serum glutamic–pyruvic transaminase (SGPT = 238 IU/L), and alkaline phosphatase (ALP = 169 IU/L) when compared with CCl4-alone treated rats (TB = 0.23 mg/Dl, SGOT = 567 IU/L, SGPT = 747 IU/L, ALP = 344 IU/L, respectively) (Dineshkumar et al., 2013).
Furthermore, E. crassipes was shown to have an effective hepatoprotective agent by virtue of its in vivo effect on liver markers and in combating oxidative stress as well, where the coadministration of the leaves aqueous extract with isoniazid in rats exhibited a 46% reduction in malondialdehyde level with concomitant elevation in the total antioxidant value of the plasma (21%). Furthermore, E. crassipes leaf aqueous extract at 400 mg/kg restored the hepatic marker levels in the serum, like alkaline phosphatase (69.22%), SGOT (29%), SGPT (62.31%), creatinine (108.80%), complete bilirubin (48.95%), and hemoglobin (65.69%) (Kumar et al., 2014). Therefore, rat models of liver injury should be investigated more to confirm the effect of E. crassipes as a liver protector agent.
Antitumor/Cytotoxic Activities
E. crassipes is known to contain some therapeutic compounds such as alkaloids and terpenoids that display anticancer properties (Aboul-Enein et al., 2014). The antitumor activity of 50% methanolic extract of E. crassipes at different doses showed a good response against melanoma tumor–bearing hybrid mice (Ali et al., 2009). The crude methanolic extract of the whole plant also revealed a notable potency against MCF-7, HeLa cells, EACC, and HepG2 cell lines with IC50 values of 1.2 ± 0.2, 1.6 ± 0.5, 6.04 ± 0.5, and 7.6 ± 1.5 μg/ml, respectively, compared to doxorubicin, a standard drug that revealed 0.28 μg/ml for HeLa and 0.42 μg/ml for both MCF-7 and HepG2 cell lines (Aboul-Enein et al., 2014). The aqueous leaf extract of E. crassipes displayed 44% inhibition against the NCI-H322 cell line and 20–31% cytotoxic activity against the T47D cell line. However, A549 and PC3 cell lines displayed resistance to E. crassipes extracts (Kumar et al., 2014). Di-amino-di-nitro-methyl dioctyl phthalate (73) and 9-(2′,2′-dimethyl-propanoilhydrazono)-2,7-bis-[2-(diethylamino)-ethoxy]fluorene (74) showed a different cytotoxic activity to different extents (Aboul-Enein et al., 2014; Mtewa et al., 2018). However, no experiments using in vivo cancer models were investigated. Thus, preclinical and clinical studies are required to assess the safety and efficacity of bioactive compounds.
Antioxidant Activities
E. crassipes induces substantial antioxidant activities, and it is confirmed to be a great source of natural antioxidants (Lalitha and Jayanthi, 2012). The plant is a source of many compounds with radical scavenging activity, such as phenolic acids, sterols, terpenoids, and other metabolites with high antioxidant activity (Tyagi and Agarwal, 2017a).
Ethanol extracts from the leaves exerted robust Fe2+ chelating activity. Meanwhile, the ethanolic extract of the flowers with a high content of phenolic compounds exhibited a substantial reducing power and radical scavenging activity (Surendraraj et al., 2013). In addition, the antioxidant properties of the methanolic crude extract of the whole plant and its isolated compounds, the alkaloids and terpenoids derivatives, were studied using the 2,2-diphenyl-1-picrylhydrazyl radical (DPPH) scavenging activity. As results the crude extract showed a good antioxidant activity while some compounds such as 1,2-benzene dicarboxylic acid, dioctyl ester (131), 1,2-benzene dicarboxylic acid, diisooctyl ester (132), 3-methyl-phenyl)-phenylmethanol (133), 4-(diethylamino)-alpha-[4-(diethylamino) phenyl] (134), and 9-(2′,2′-dimethyl-propanoilhydrazono)-2,7-bis-[2-(diethylamino)-ethoxy]fluorene (74) recorded moderate activities with IC50 ranging between 97.0 ± 5.4 and 97.4 ± 2.7 μg/ml (Aboul-Enein et al., 2014). The high antioxidant potential of the methanolic crude extract could be explained by virtue of the synergistic activities of all bioactive compounds (Aboul-Enein et al., 2014). E. crassipes extracts have shown encouraging antiaging effects, as determined through DNA damage inhibition and DPPH radical scavenging assays. There was a pronounced increase in the DNA damage inhibition and DPPH radical scavenging ability with an increase in the concentration of ethyl acetate extracts of the plant (Lalitha and Jayanthi, 2014). Moreover, the highest radical scavenging activity was observed in the petiole with an IC50 value = 6.411 ± 0.46 mg/ml as compared with IC50 = 0.516 ± 0.22 mg/ml obtained by the reference compound—the gallic acid (Tyagi and Agarwal, 2017b).
The methanolic extract of E. crassipes showed good DPPH radical scavenging activity with a maximum inhibition of 78% at 250 μg/ml, while in hydrogen peroxide scavenging activity, the maximum inhibition was 80% observed at 250 μg/ml; ascorbic acid, a standard antioxidant drug demonstrated a maximum inhibition of 69% and 68% in both tests at 100 μg/ml, respectively (Sunitha et al., 2018). In the same line, methanol, n-hexane, and carbon tetrachloride extracts of the leaves demonstrated free radical scavenging activity with IC50 of 0.018, 0.387, and 1.03 μg/ml, respectively (Islam, 2018). Recently, the antioxidant properties of the leaf protein hydrolysates indicated excellent antioxidant activities, especially the two peptides that have shown high radical scavenging activities with 86.37% of superoxide anion radical scavenging activity at 1 mg/ml and 56.51% of ABTS cation radical scavenging activity at 100 μg/ml (Zhang et al., 2018). However, quercetin 7-methyl ether (30) isolated from the whole plant exhibited weak antioxidant activities using DPPH method, with an IC50 = 254.66 μg/ml compared with quercetin (IC50 = 23.24 mg/ml) (Elvira et al., 2018). Thus, additional in vivo studies are required to confirm the important effect demonstrated by in vitro studies, to determine the molecular mechanisms of the extracts and the bioactive compounds found in E. crassipes.
Antimicrobial Activities
Many extracts of E. crassipes demonstrated antibacterial and antifungal activities (Table 4).
In Ethiopia, it has been used for the preparation of crude medicine for treating numerous kinds of virulent diseases related to bacterial infections (Kiristos et al., 2018). In fact, the presence of saponins in the leaves makes them a good candidate, with notable biopotency, as an antimicrobial agent. Gutiérrez-Morales et al. (2017) revealed the potential of E. crassipes leaf extract in combating staphylococcal infections, against methicillin-resistant S. aureus (MRSA) found in cattle and Coagulase-negative staphylococci (CoNS) in rabbits. The inhibition of the growth (61.7–68.6%) and division of bacteria could be due to the saponins glycosides and their aglycones (Gutiérrez-Morales et al., 2017).
E. crassipes displayed antibacterial activities against S. faecalis, E. coli, and S. aureus. Meanwhile, developments of A. niger, A. flavus, and C. albicans were repressed by the plant through crude extract or its fractions (Shanab et al., 2010). The water extract of the leaves demonstrated antimicrobial activity as well (zone of inhibition, 8–22 mm) against Bordetella bronchiseptica, Proteus vulgaris, and Salmonella typhi (Kumar et al., 2014).
In addition, the antibacterial activities of silver nanoparticles, synthesized biologically from the extract of E. crassipes, were checked against selected gram-positive and gram-negative bacteria, and significant zones of inhibition were observed (ZOI ranged between 13 and 18 mm) (Kiruba Daniel et al., 2012; Thombre et al., 2014). Joshi and Kaur (2013) investigated the antimicrobial activity of hydroalcoholic and ethanolic extracts on E. coli, S. epidermidis, P. aeruginosa, and B. subtilis (Table 4). The antifungal effects of the shoots and leaves of the ethanol extracts were evaluated against two fungi, A. fumigates and M. ruber, employing the disk diffusion method. They revealed notable activity (ZOI = 11 and 12 mm, respectively) toward all the tested organisms comparable to the standard cotrimaxozole (ZOI = 16 and 18 mm) (Thamaraiselvi and Jayanthi, 2012). Furthermore, the antifungal and antibacterial effects of different extracts of the plant against seven phytopathogenic fungi and 11 clinical bacteria showed that the most susceptible organisms were K. pneumoniae, S. typhi, S. rolfsii, and F. moniliforme. The methanolic fraction was more effective (54.45%) against the bacterial strains as compared to the cold aqueous extract (Baral and Vaidya, 2011). It has been noted that aqueous extracts of the leaves contained active compounds such as chlorogenic acid, alkaloids, flavonoids, sterols, anthocyanins, and quinones, which significantly improved resistance against pathogen Lactococcus garvieae in prawn (Jayanthi et al., 2011; Chang and Cheng, 2016). The ethyl acetate extracts prepared from the stems showed significant antimicrobial activity at 2 mg against S. aureus and S. typhi (activity index = 0.21 and 0.23, respectively). While the ethyl acetate extracts of the leaves, at the same concentration, were only active against S. typhi with an activity index of 0.24 (Hossain et al., 2018). The n-butyl alcohol extract exhibited antimicrobial activities against some bacteria including E. coli, B. cereus, L. casei, and B. subtilis (MIC = 16 μg/ml) and antifungal activity against six pathogenic fungi: A. flavus, A. niger, A. alternata, C. gloeosporioides, C. albicans, and F. solani (minimum inhibitory concentration ranged between 8 and 32 μg/ml) (Haggag et al., 2017). Concerning staphylococcal contaminations, E. crassipes showed a high potency against MRSA found in cows, oxacillin-sensitive S. aureus (SOSA), and coagulase-negative S. epidermidis (CoNS) present in bunnies (Gutiérrez-Morales et al., 2017). The in silico antibacterial activity of stigmasterol, 1-monolinoleoylglycerol trimethylsilyl ether, 17-pentatriacontene, and octasiloxane phytocompounds from E. crassipes leaves was assessed by the inhibition of AprX enzyme through molecular docking. The results showed that the phytocompounds are strong inhibitors of AprX enzyme with better degrees of docking and interaction analysis (Kumar et al., 2018b). Moreover, the antibacterial activity of iron oxide nanoparticles (FeNPs) synthesized using the leaf extract of the plant was determined using well diffusion method. The FeNPs showed good antibacterial activity with the highest zone of inhibition at 100 μg/ml against Staphylococcus aureus (23.3 mm) and Pseudomonas fluorescens (22.6 mm) (Jagathesan and Rajiv, 2018).
E. crassipes water leaf extract showed totally bacteriostatic and bactericidal activities at concentrations of 6.25–100%, against Aggregatibacter actinomycetemcomitans, a gram-negative bacterium and the major cause of aggressive periodontitis, at a minimal concentration of 1.56% (Afidati et al., 2019). From all studies, it can be concluded that the process of extractions and the type of solvent used could affect the microbial activity of E. crassipes.
Wound Healing Activity
E. crassipes could be used in cosmeceutical preparations because of its wound healing efficiency.
In Nigeria, the plant is used for skin care applications (Abd El-Ghani, 2016). Moreover, the leaf extract of the plant combined with turmeric and rice flour were used to treat eczema. This activity is due to the significant levels of vitamin C reported in the plant (Sharma et al., 2020).
The methanol extract of E. crassipes leaves was formulated as an ointment using 10 and 15% of leaf extracts and had significantly improved wound contraction potential compared to the control due to the presence of phenolic compounds (Ali et al., 2010). In the same line, the plant extracts demonstrated encouraging antiaging effects through DNA damage inhibition. The ethyl acetate extract of the E. crassipes plant, in combination with musk and lemon, was formulated as a cream and revealed 8–11% tyrosinase inhibition with skin whitening effects. Furthermore, the inhibition of DNA damage was correlated with the increase in concentration of the ethyl acetate extracts (Lalitha and Jayanthi, 2014). More attention and effort should be given to the investigation of the wound healing effect and the underlining molecular mechanisms for promising cosmeceutical industry prospects.
Other Biological Activities
Larvicidal Activity
E. crassipes displayed effective larvicidal activity in which the crude root extract showed effects on Chironomus ramosus eggs and larvae in addition to the toxic potential of the acetone extract toward the two pests Achaea janata (LD50 > 100 mg/21 m2/larva) and Spodoptera litura (Fab.) (LD50 = 93 mg/21 m2/larva) (Devanand and Rani, 2008). The ethanol extract of E. crassipes leaves and shoot showed higher larvicidal activity against C. quinquefasciatus (LC50 = 71.43, 94.68, 120.42, and 152.15 ppm) compared to other solvent extracts. This activity might be due to the presence of metabolites like anthraquinones, alkaloids, and flavonoids (Jayanthi et al., 2012). Sterols, sitosterol, have been reported to possess larvicidal activity (Rahuman et al., 2008). The crude ethyl acetate, hexane, methanol, and aqueous leaf extracts were tested for larvicidal effects against the early fourth instar larvae of C. quinquefasciatus. The results showed that hexane and methanol extracts were the most effective at doses of 62.5 and 500 mg/L with an LC50 value of 80.54 and 137.50 mg/L, respectively (Annie et al., 2015). Furthermore, the effect of the plant infusions on mosquito attractiveness and stimulation of oviposition was investigated, and the results suggested that the plant emits volatile chemicals, such as terpenoids and fatty acid derivatives that attract A. aegypti and A. quadrimaculatus, and stimulates the egg rafts position of C. quinquefasciatus (Turnipseed et al., 2018).
Allelopathic Effect
The extracts from the sterilized culture of E. crassipes were tested for their inhibition of Chlamydomonas reinhardtii. At a low concentration, the extract did not inhibit the growth of C. reinhardtii. However, inhibition increased at higher concentrations of the exudate since 100 µL of the extract exhibited 100% inhibition (Sun et al., 1990). Sterols, isolated from the ethyl acetate extract of the plant, were tested for their phytotoxic activity on radish root growth. 4α-methyl-5α-ergosta-8,24(28)-diene-3β,4β-diol and 4α-methyl-5α-ergosta-8,14,24(28)-triene-36,48-diol inhibited, respectively, 40% and 30% of radish root elongation at 6 µmol (DellaGreca et al., 1991).
Linoleic acid (44), glycerol-1,9-12(ZZ)-octadecadienoic ester (125), and N-phenyl-2-naphthylamine (126) isolated from the acetone extract of the roots showed a stronger anti-algal effect than the common algaecide CuSO4 (Shanyuan et al., 1992). The crude extract of E. crassipes and its fractions exhibited some anti-algal activity against the green microalgae: Dictyochloropsis splendida and Chlorella vulgaris. This activity was high against Chlorella vulgaris (ZOI = 18–33 mm) and could be attributed to the presence of phthalate derivatives and alkaloids (Shanab et al., 2010).
Wu et al. (2012) investigated the allelopathic effect of the plant against Microcystis aeruginosa using coexistence assay. As a result, the growth of the blue-green algae root system was significantly inhibited by the hydroalcoholic extract of the plant. By contrast, no allelopathic effect of the plant on spinach growth was noticed (Barman et al., 2006).
Moreover, the phytotoxic effect of the leaves extract of the plant was assessed against Mimosa pigra (an invasive weed) and Vigna radiata (a crop species). The results of the biochemical parameters demonstrated the allelopathy activity of the plant extract against the speed germination of M. pigra and V. radiata. The H2O2 content of the root tissues of M. pigra and V. radiata seeds increased 4.3 and 3.8 folds, respectively, with 5% of the extract. Furthermore, the 5% extract reduced the MDA content of the non-pregerminated and pregerminated seedlings by 18% and 44%, respectively, and resulted in the inhibition of 66% and 59% in the soluble POD activities (Chai et al., 2013). However, it could be interesting to investigate the effect of natural compounds isolated from E. crassipes as herbicides, since few research have been conducted. Moreover, further research is required on the physiological and ecological mechanisms of allelopathy for its worldwide application in agricultural production.
Insecticidal Activity
Few studies have demonstrated the insecticidal potential of E. crassipes extracts against household insects (Hassan, 2013; Lenora and Senthilkumar, 2017). The antifeedant potential of plant extracts at 2% varied against Tobacco caterpillar, with 57.8% in hexane extract and 35.9% in methanol extract (Lenora and Senthilkumar, 2017). This activity could be related to the presence of terpenoids. These results confirm the strong insecticidal activity of the plant. Future research will further explore the in-depth mechanistic effect of the plant and its bioactive compounds, to highlight its potential as natural, plant-derived pesticide for the management of plant pests.
Immunostimulant Effect
E. crassipes has been utilized as an immunostimulant for protection against viral, bacterial, and fungal diseases related to aquaculture. Chang et al. (2013) stated that the extract of the plant, at 2 and 3 g/kg, enhanced immune responses and resistance of prawn Macrobrachium rosenbergii against Lactococcus gravieae by 39.1% and 52.2%, respectively. Moreover, different strategies using the water extracts of E. crassipes leaves were incorporated into the diet of the prawn Macrobrachium rosenbergii as an immunostimulant against Lactococcus gravieae. As a result, the long-term administration of the infusion of the plant (2–20 g/kg) had increased innate immunity by 88.4% and resistance against the pathogen by 68.5% (Chang and Cheng, 2016). The dietary administration of E. crassipes water extract improved immunity (higher immune parameters such as LYZ, Ig, ACH50, and RBA with more than 1000 U/mL) and enhanced the resistance of rainbow trout Oncorhychus mykiss against Streptococcus iniae by 49.6% (Rufchaei et al., 2020).
Animal Feed Formulation
E. crassipes is rich in protein, vitamins, and minerals and is used as duck feed. In Indonesia, China, Philippines, and Thailand, the plant serves as a high-quality feedstock for some nonruminant animals and poultry, and in fishery. The plant biomass is also commonly used as forage for cattle, as basal feed resource or supplement to a diet consisting of sugarcane, molasses, and cereal straw, as it contains adequate minerals that are sufficient for maintenance and production requirements (Hossain et al., 2015; Tham, 2016).
Patents Including E. crassipes (Mart.) Solms
Several inventions have focused on exploring some potential ways to produce high value-added products from E. crassipes (Mart.). Cumulative increases in the number of patents published in the last few years clearly justifies the importance of the weed in the treatment of various disorders and as a source for new therapeutic agents. As shown in Table 5, these patents have highlighted the use of the different plant parts in various applications such as antiaging, antioxidant, anti-microbial, anti-inflammatory, among others. In general, several patents found in the literature have disclosed the use of E. crassipes in the cosmetic industries, combining a traditional formula and using modern techniques of extractions to guarantee strong effects. The invention by Wang (2015) and Cui (2015) provided methods of formulation of hand and herb creams, respectively. The hand cream prepared from the plant can accelerate skin healing from secondary infection during the treatment period. The cream cooperates with the immune system to eliminate inflammation, relieve itches, and remove edema. While the herb cream is prepared by using E. crassipes with other herbal medicines through a modern technology, Cui (2015) has reported that the cream is suitable for preventing skin infections caused by fungi and bacteria. In addition, the invention by Leconte and Rossignol-Castera (2014) has described a method to prepare a novel cosmetic composition using the lipophilic extract of water hyacinth for moisturizing the skin, and to maintain and restore the hydration of the skin. Other inventions are related to the utilization of E. crassipes in medicine and pharmacology. The invention by Yu et al. (2020) introduced a pharmaceutical composition for use in the treatment of inflammation. They reported that triterpenoid improves anti-inflammatory activity and antioxidant capacity, with high industrialization value.
Conclusion and Perspectives
This comprehensive review on the phytochemical composition and pharmacological/biological activities of the plant was done to assess the chemical composition and value-added applications of E. crassipes aiming to highlight the plant's potential to enhance its limited pharmaceutical applications in Africa, especially in Ethiopia.
In this review, various constituents of the plant have been identified for a multitude of applications. The results of multiple phytochemical studies rely on the isolation and identification of various phytocompounds such as polyphenols, flavonoids, sterols, alkaloids, among other secondary metabolites. Phytosterols and terpenoids, considered as major compounds, could be used to provide value-added compounds for the food and pharmaceutical industries. Moreover, the physicochemical processes have been used to produce other value-added products from E. crassipes biomass, such as furfural, xylitol, enzymes, polymers, and composites and have been applied in distinct fields of applications. In this line, it will be interesting to study various strategies using combined processes for by-products production at the industrial scale. In addition, pharmacological and biological properties of E. crassipes have been discussed in detail. Different extracts and bioactive compounds isolated from the plant showed anticancer ability against various cancer cell lines. In addition, different studies witnessed the anti-inflammatory, antioxidant, antibacterial, and antifungal activities of E. crassipes extracts. Furthermore, several patents have described the pharmacological effect of the plant, but clinical applications are still rare and should be further evaluated. Since most of the studies those reported the potential effect of E. crassipes on health are animal-based studies, pharmacological findings need to be supported by the mechanisms. Other studies showed the use of E. crassipes extracts in wound healing. The plant has demonstrated potential effects in antiaging. Recent innovations targeted the development of new formulations in related fields for the standardization and validation of the plant as an antiaging agent. However, the plant requires further attention for the isolation of bioactive compounds responsible for biological activities. Accordingly, it is important to further clarify the effectiveness of compounds and elucidate their toxicity for future studies.
Undoubtedly, the limitations could not be avoided in this study in terms of quality and the limited number of included studies. Concurrently, new findings could increase the present therapeutic importance of E. crassipes and promote its future uses in modern medicine. Furthermore, it is necessary to investigate the pharmacological and toxicological mechanisms of the plant and establish an effective evaluation system which could promote the development and application of this valuable resource in pharmaceutical industries.
Author Contributions
All authors listed have made a substantial, direct, and intellectual contribution to the work and approved it for publication. WBB collected the appropriate literature, analyzed the data, and drafted the manuscript. AE and FK provided helpful comments. MS revised the manuscript and provided helpful comments. MK, MH, LK and AY critically revised the manuscript. All authors approved the final version of the manuscript.
Funding
The authors are thankful to OCP and OCP Ethiopia for their assistance and financial support of this research, which is a part of the project entitled “Integrated sustainable management of the water hyacinth in Lake Tana, Ethiopia.”
Conflict of Interest
The authors declare that the research was conducted in the absence of any commercial or financial relationships that could be construed as a potential conflict of interest.
Publisher’s Note
All claims expressed in this article are solely those of the authors and do not necessarily represent those of their affiliated organizations, or those of the publisher, the editors, and the reviewers. Any product that may be evaluated in this article, or claim that may be made by its manufacturer, is not guaranteed or endorsed by the publisher.
Supplementary Material
The Supplementary Material for this article can be found online at: https://www.frontiersin.org/articles/10.3389/fphar.2022.842511/full#supplementary-material
References
Abd El-Ghani, M. M. (2016). Traditional Medicinal Plants of Nigeria: an Overview. Agric. Biol. .J .N. Am. 7 (5), 220–247. doi:10.5251/abjna.2016.7.5.220.247
Abdul Rahuman, A., Gopalakrishnan, G., Venkatesan, P., and Geetha, K. (2008). Isolation and Identification of Mosquito Larvicidal Compound from Abutilon Indicum (Linn.) Sweet. Parasitol. Res. 102, 981–988. doi:10.1007/s00436-007-0864-5
Aboul-Enein, A. M., Al-Abd, A. M., Shalaby, E., Abul-Ela, F., Nasr-Allah, A. A., Mahmoud, A. M., et al. (2011). Eichhornia crassipes (Mart) Solms: from Water Parasite to Potential Medicinal Remedy. Plant Signal. Behav. 6 (6), 834–836. doi:10.4161/psb.6.6.15166
Aboul-Enein, A. M., Shanab, S. M., Shalaby, E. A., Zahran, M. M., Lightfoot, D. A., and El-Shemy, H. A. (2014). Cytotoxic and Antioxidant Properties of Active Principals Isolated from Water Hyacinth against Four Cancer Cells Lines. BMC Complement. Altern. Med. 14 (December), 397. doi:10.1186/1472-6882-14-397
Abral, H., Dalimunthe, M. H., Hartono, J., Efendi, R. P., Asrofi, M., Sugiarti, E., et al. (2018). Characterization of Tapioca Starch Biopolymer Composites Reinforced with Micro Scale Water Hyacinth Fibers. Starch - Stärke 70 (7–8), 1700287. doi:10.1002/star.201700287
Adelodun, A. A., Hassan, U. O., and Nwachuckwu, V. O. (2020). Environmental, Mechanical, and Biochemical Benefits of Water Hyacinth (Eichhornia crassipes). Environ. Sci. Pollut. Res. Int. 27, 30210–30221. doi:10.1007/s11356-020-09221-1
Arifkhodzhaev, A. O., and Shoyakubov, R. S. (1995). Polysaccharides of Eichhornia crassipes and Pistia stratiotes. Chem. Nat. Compd. 31 (4), 521–522.
Afidati, Y. I., Savitri, I. J., and Krismariono, A. (2019). Inhibition Activity of Water Hyacinth Leaf Extract (Eichhornia crassipes) against Aggregatibacter Actinomycetemcomitans. Asian J. Pharm. Clin. Res. 12 (6), 122–125. doi:10.22159/ajpcr.2019.v12i6.33162
Ahmed, H. R., Badawi, H., Ali, S. A., and Fayez, M. (2018). The Floating Aquatic Water Hyacinth (Eichhornia crassipes) Is a Multi-Uses Macrophyte for Fuel Ethanol Production and Pathogen Control. Int. J. Eng. Sci. 8 (12), 19406–19418.
Ali, H., Lata, N., Ahi, J., and Ganesh, N. (2010). Evaluation of Wound-Healing Activity of Eichornia Crassipes: A Novel Approach. Drug Invent 2 (3), 1.
Ali, H., Patel, M., Ganesh, N., and Ahi, J. (2009). The World’s Worst Aquatic Plant as a Safe Cancer Medicine Antitumor Activity on Melanoma Induced Mouse by Eichornia Crassipes : In Vivo Studies. J. Pharm. Res. 2 (7), 1365–1366.
Amriani, F., Salim, F. A., Iskandinata, I., Khumsupan, D., and Barta, Z. (2016). Physical and Biophysical Pretreatment Of Water Hyacinth Biomass For Cellulase Enzyme Production. Chem. Biochem. Eng. Q. 30 (2), 237–244.
Anjaneyalu, Y., Gowda, D., and Neelisiddiah, B. (1983). Structural Features of a Polysaccharide from the Mucin of Water Hyacinth. Phytochemistry 22 (9), 1961–1963. doi:10.1016/0031-9422(83)80023-5
Annie, S. W., Raveen, R., Paulraj, M. G., Samuel, T., and Arivoli, S. (2015). Screening of Eichhornia crassipes (Mart.) Solms (Pontederiaceae) Crude Leaf Extracts for Larvicidal Efficacy against the Filarial Vector Culex quinquefasciatus Say (Diptera: Culicidae). Int. J. Mosq. Res. 2, 43–48.
Arismawati, A., Ulfah, N., and Bargowo, L. (2021). Inhibition Activity of Water Hyacinth Leaf Extract (Eichornia Crassipes) to the Growth of Subgingival Plaque Bacteria Colony. Mlu 21 (1), 552–556. doi:10.37506/mlu.v21i1.2369
Asrofi, M., Abral, H., Kasim, A., Pratoto, A., Mahardika, M., and Hafizulhaq, F. (2018). Mechanical Properties of a Water Hyacinth Nanofiber Cellulose Reinforced Thermoplastic Starch Bionanocomposite: Effect of Ultrasonic Vibration during Processing. Fibers 6 (2), 40. doi:10.3390/fib6020040
Asrofi, M., Abral, H., Kasim, A., and Pratoto, A. (2017). XRD and FTIR Studies of Nanocrystalline Cellulose from Water Hyacinth (Eichornia Crassipes) Fiber. Jmnm 29, 9–16. doi:10.4028/www.scientific.net/jmnm.29.9
Balasubramanian, D., Arunachalam, K., Das, A. K., and Arunachalam, A. (2012). Decomposition and Nutrient Release of Eichhornia crassipes (Mart.) Solms. Under Different Trophic Conditions in Wetlands of Eastern Himalayan Foothills. Ecol. Eng. 44, 111–122. doi:10.1016/j.ecoleng.2012.03.002
Baral, B., Vaidya, G. S., and Bhattarai, N. (2012). Bioactivity and Biochemical Analysis of Water Hyacinth (Eichhornia crassipes). Botanica Orientalis 8, 33–39. doi:10.3126/botor.v8i0.5556
Baral, B., and Vaidya, G. S. (2011a). Bio-assay and Phytochemical Screening of Water Hyacinth of Nepalese Wetland 3, 80–86.
Baral, B., and Vaidya, G. S. (2011b). Biological and Chemical Assessment of Water Hyacinth (Eichhornia crassipes (Mart.) Solms.) of Phewa Lake, Nepal. Sci. World 9 (9), 57–62. doi:10.3126/sw.v9i9.5520
Barman, K. K., Ansari, N. G., and Dubey, M. (2006). Effect of Water Hyacinth on Biomass Yield of Spinach and Nutrient Availability. J. Indian Soc. Soil Sci. 54 (1), 75–79.
Bochkov, D. V., Sysolyatin, S. V., Kalashnikov, A. I., and Surmacheva, I. A. (2012). Shikimic Acid: Review of its Analytical, Isolation, and Purification Techniques from Plant and Microbial Sources. J. Chem. Biol. 5 (1), 5–17. doi:10.1007/s12154-011-0064-8
Bodo, R., Azzouz, A., and Hausler, R. (2004). Antioxidative Activity of Water Hyacinth Components. Plant Sci. 166 (4), 893–899. doi:10.1016/j.plantsci.2003.12.001
Cardoso, S. F., Lopes, L. M. X., and Nascimento, I. R. (2014). Eichhornia crassipes: an Advantageous Source of Shikimic Acid. Revista Brasileira de Farmacognosia 24, 439–442. doi:10.1016/j.bjp.2014.08.003
Carreño Sayago, U. F., and Rodríguez, C. (2018). Design and Construction of a Biohydrogen and Bioethanol Production System from the Biomass of the Eichhornia crassipes. doi:10.20944/preprints201805.0393.v1
C.G. Kiruba Daniel, S., Nehru, K., Sivakumar, M., and Pitchumani, K. (2012). Rapid Biosynthesis of Silver Nanoparticles Using Eichornia Crassipes and its Antibacterial Activity. Cnano 8 (1), 125–129. doi:10.2174/1573413711208010125
Chai, T. T., Jiang-Chin, N., and Wong, F. C. (2013). Herbicidal Potential of Eichhornia crassipes Leaf Extract against Mimosa Pigra and Vigna Radiata. Int. J. Agric. Biol. 15 (5), 835–842.
Chang, C.-C., and Cheng, W. (2016). Multiple Dietary Administrating Strategies of Water Hyacinth (Eichhornia crassipes) on Enhancing the Immune Responses and Disease Resistance of Giant Freshwater Prawn,Macrobrachium Rosenbergii. Aquac. Res. 47 (1), 140–152. doi:10.1111/are.12477
Chang, C. C., Tan, H. C., and Cheng, W. (2013). Effects of Dietary Administration of Water Hyacinth (Eichhornia crassipes) Extracts on the Immune Responses and Disease Resistance of Giant Freshwater Prawn, Macrobrachium Rosenbergii. Fish. Shellfish Immunol. 35 (1), 92–100. doi:10.1016/j.fsi.2013.04.008
Chantiratikul, P., Meechai, P., and Nakbanpotec, W. (2009). Antioxidant Activities and Phenolic Contents of Extracts from Salvinia Molesta and Eichornia Crassipes. Res. J. Biol. Sci. 4 (10), 1113–1117.
Cui, Y. (2015). Eichhornia crassipes Herb Cream. Patent No CN104415178A. China: Qingdao Medical Prevention Disinfection Professional Technology Center.
Dantas-Santos, N., Gomes, D. L., Costa, L. S., Cordeiro, S. L., Costa, M. S., Trindade, E. S., et al. (2012). Freshwater Plants Synthesize Sulfated Polysaccharides: Heterogalactans from Water Hyacinth (Eicchornia Crassipes). Int. J. Mol. Sci. 13 (1), 961–976. doi:10.3390/ijms13010961
Della Greca, M., Lanzetta, R., Molinaro, A., Monaco, P., and Previtera, L. (1992). Phenalene Metabolites from Eichhornia crassipes. Bioorg. Med. Chem. Lett. 2 (4), 311–314. doi:10.1016/s0960-894x(01)80207-9
Della Greca, M., Monaco, P., and Previtera, L. (1991). New Oxygenated Sterols from the weed Eichhornia crassipes Solms. Tetrahedron 47 (34), 7129–7134. doi:10.1016/s0040-4020(01)96166-6
DellaGreca, M., Previtera, L., and Zarrelli, A. (2008). Revised Structures of Phenylphenalene Derivatives from Eichhornia crassipes. Tetrahedron Lett. 49 (20), 3268–3272. doi:10.1016/j.tetlet.2008.03.072
DellaGreca, M., Previtera, L., and Zarrelli, A. (2009). Structures of New Phenylphenalene-Related Compounds from Eichhornia crassipes (Water Hyacinth). Tetrahedron 65 (39), 8206–8208. doi:10.1016/j.tet.2009.07.069
Deng, L., Geng, M., Zhu, D., Zhou, W., Langdon, A., Wu, H., et al. (2012). Effect of Chemical and Biological Degumming on the Adsorption of Heavy Metal by Cellulose Xanthogenates Prepared from Eichhornia crassipes. Bioresour. Technolbioresour. Technol. 107, 41–45. doi:10.1016/j.biortech.2011.12.023
Dersseh, M. G., Melesse, A. M., Tilahun, S. A., Abate, M., and Dagnew, D. C. (2019). “Water Hyacinth: Review of its Impacts on Hydrology and Ecosystem Services-Lessons for Management of Lake Tana,” in Extreme Hydrology and Climate Variability. Editors A. M. Melesse, W. Abtew, and G. Senay (Amsterdam, The Netherlands: Elsevier), 237–251. doi:10.1016/b978-0-12-815998-9.00019-1
Deshpande, P., Nair, S., and Khedkar, S. (2009). Water Hyacinth as Carbon Source for the Production of Cellulase by Trichoderma Reesei. Appl. Biochem. Biotechnol. 158 (3), 552–560. doi:10.1007/s12010-008-8476-9
Devanand, P., and Rani, P. U. (2008). Biological Potency of Certain Plant Extracts in Management of Two Lepidopteran Pests of Ricinus communis L. J. Biopest. 1 (2), 170–176.
Dineshkumar, G., Rajakumar, R., Mani, P., and Johnbastin, T. M. M. (2013). Hepatoprotective Activity of Leaves Extract of Eichhornia crassipes against CCl4 Induced Hepatotoxicity Albino Rats. Int. J. Pure Appl. Zool. 1 (3), 209–212.
Elvira, K., Fachriyah, E., and Kusrini, D. (2018). Isolation of Flavonoid Compounds from Eceng Gondok (Eichhornia crassipes) and Antioxidant Tests with DPPH (1,1-Diphenyl-2-Picrylhydrazyl) Method. J. Kim. Sains Apl. 21 (4), 187–192. doi:10.14710/jksa.21.4.187-192
Farheen, M., Arman, S., Hussaini, R., Kouser, A., and Pharm, M. (2015). Phytochemical Evaluation and Pharmacological Screening of Ethanolic Leaf Extracts of Eichhornia crassipes and Nelumbo nucifera for Neuropharmacological Activity in Psychoneurosis Induced Mice. World J. Pharm. Pharm. Sci. 4 (12), 874–904.
Fileto-Pérez, H. A., Rutiaga-Quiñones, O. M., Sytsma, M. D., Lorne, I. M., Luo, W., Pankow, J. F., et al. (2015). GC/MS Analysis of Some Extractives from. Eichhornia crassipes. Bioresources 10 (4), 7353–7360.
Flores Ramirez, N., Sanchez Hernandez, Y., Cruz de Leon, J., Vasquez Garcia, S. R., Domratcheva Lvova, L., and Garcia Gonzalez, L. (2015). Composites from Water Hyacinth (Eichhornea Crassipe) and Polyester Resin. Fibers Polym. 16, 196–200. doi:10.1007/s12221-015-0196-5
G, A., S, B., K, M. P., G, S., and U, G. P. (2020). Extraction and Molecular Characterization of Biological Compounds from Water Hyacinth. J. Med. Plants Stud. 8 (5), 14–19. doi:10.22271/plants.2020.v8.i5a.1189
Ghabbour, E. A., Davies, G., Lam, Y. Y., and Vozzella, M. E. (2004). Metal Binding by Humic Acids Isolated from Water Hyacinth Plants (Eichhornia crassipes [Mart.] Solm-Laubach: Pontedericeae) in the Nile Delta, Egypt. Environ. Pollut. 131 (3), 445–451. doi:10.1016/j.envpol.2004.02.013
Goswami, P. C., Nag, B., Sharma, A. K., Borthakur, A., Singh, H. D., and Baruah, J. N. (1983). Water Hyacinth as a Prospective Source of Stigmasterol. Curr. Sci. 52 (17), 806–808.
Greca, M. D., Molinaro, A., Monaco, P., and Previtera, L. (1993). Degraded Phenalene Metabolites in Eichhornia crassipes. Nat. Prod. Lett. 1 (4), 233–238.
Guna, V., Ilangovan, M., Anantha Prasad, M. G., and Reddy, N. (2017). Water Hyacinth: a Unique Source for Sustainable Materials and Products. ACS Sustainable Chem. Eng. 5, 4478–4490. doi:10.1021/acssuschemeng.7b00051
Gutiérrez-Morales, A., Velázquez-Ordoñez, V., Khusro, A., Salem, A. Z. M., Estrada-Zúñiga, M. E., Salem, M. Z. M., et al. (2017). Anti-staphylococcal Properties of Eichhornia crassipes, Pistacia Vera, and Ziziphus Amole Leaf Extracts: Isolates from Cattle and Rabbits. Microb. Pathogenesis. 113, 181–189. doi:10.1016/j.micpath.2017.10.015
Haggag, M. W., Abou El Ella, S. M., and Abouziena, H. F. (2017). Phytochemical Analysis, Antifungal, Antimicrobial Activities and Application of Eichhornia crassipes against Some Plant Pathogens. Planta Daninha 35, 1–11. doi:10.1590/S0100-83582017350100026
Hamid, H. H., Ghaima, K. K., and Najem, A. M. (2013). Phytochemical, Antioxidant and Antibacterial Activities of Some Extracts of Water Hyacinth (Eichhornia crassipes) Leaves. Int. J. Adv. Pharm. Res. 4, 1847–1851.
Hassan, H. (2013). The Effect of Water Hyacinth, Eichhornia crassipes, and King-Bo Compound on Some Biological and Biochemical Aspects on Cotton Leaf Worm Spodoptera Littoralis (Boisd). Egypt. Acad. J. Biol. Sci. F. Toxicol. Pest Control. 5 (1), 33–45. doi:10.21608/EAJBSF.2013.17270
Hossain, J., Khan, A., and Uddin, M. A. (2018). Antimicrobial Efficacy and Phytochemical Analysis of Three Aquatic Plant Species in Bangladesh. Bangla. J. Microbiol. 35 (1), 7–11. doi:10.3329/bjm.v35i1.39797
Hossain, M. E., Sikder, H., Kabir, M. H., and Sarma, S. M. (2015). Nutritive Value of Water Hyacinth (Eichhornia crassipes). Online J. Anim. Feed Res. 5, 40–44.
Hussain, Z., Khan, K. M., Perveen, S., Zaman, K., Hayat, G., Karim, A., et al. (2015). The Long Chain Alcohols of the N-Hexane Fraction of Water Hyacinth (Eichhornia crassipes): Extraction, Estimation, GC-MS Analysis and Antimicrobial Activity. J. Chem. Soc. Pak. 37 (1), 144–149.
Ilo, O. P., Simatele, M. D., Nkomo, S. p. L., Mkhize, N. M., and Prabhu, N. G. (2020). The Benefits of Water Hyacinth (Eichhornia crassipes) for Southern Africa: A Review. Sustainability 12, 9222. doi:10.3390/su12219222
Islam, S. (2018). In Vitro evaluation of Thrombolytic and Antioxidant Scavenging Activity of Eichhornia crassipes. SEU J. Eng. Sci. 12 (2), 1.
Jagathesan, G., and Rajiv, P. (2018). Biosynthesis and Characterization of Iron Oxide Nanoparticles Using Eichhornia crassipes Leaf Extract and Assessing Their Antibacterial Activity. Biocatal. Agric. Biotechnol. 13, 90–94. doi:10.1016/j.bcab.2017.11.014
Jayanthi, P., Lalitha, P., and Aarthi, N. (2012). Larvicidal and Pupicidal Activity of Extracts and Fractionates of Eichhornia crassipes (Mart.) Solms against the Filarial Vector Culex quinquefasciatus Say. Parasitol. Res. 111 (5), 2129–2135. doi:10.1007/s00436-012-3061-0
Jayanthi, P., Lalitha, P., and Sripathi, S. K. (2011). Phytochemical Investigation of the Extracts of Eichhornia crassipes and its Solvent Fractionates. J. Pharm. Res. 4 (5), 1405–1406.
Jayanthi, P., Lalitha, P., Sujitha, R., and Thamaraiselvi, A. (2013). Anti-inflammatory Activity of the Various Solvent Extracts of Eichhornia crassipes (Mart.) Solms. Int. J. Pharmtech Res. 5 (2), 641–645.
Jin, Z. H., Zhuang, Y. Y., Dai, S. G., and Li, T. L. (2003). Isolation and Identification of Extracts of Eichhornia crassipes and Their Allelopathic Effects on Algae. Bull. Environ. Contam. Toxicol. 71 (5), 1048–1052. doi:10.1007/s00128-003-0226-7
Joshi, M., and Kaur, S. (2013). In Vitro evaluation of Antimicrobial Activity and Phytochemical Analysis of Calotropis Procera, Eichhornia crassipes and Datura Innoxia Leaves. Asian J. Pharm. Clin. Res. 6 (Suppl. 5), 25–28.
Preethi, K., and Umesh, V. M. (2015). Water Hyacinth: A Potential Substrate For Bioplastic (Pha) Production Using Pseudomonas aeruginosa. Int. J. Appl. Res. 1 (11), 349–354.
Kalhorinia, S., Goli, J. K., Yadav, K. S., Naseeruddin, S., and Rao, L. V. (2014). Xylitol Production from Water Hyacinth (Eichhornia crassipes) by Candida tropicalis Y-27405. Biosci. Biotechnol. Res. Asia 11 (2), 427–434. doi:10.13005/bbra/1291
Karmakar, M., and Ray, R. (2011). Statistical Optimization of FPase Production from Water Hyacinth using Rhizopus oryzea PR 7. J. Biochem. Technol. 3 (1), 225–229.
Khalid, S., Shaheen, S., Hussain, K., Shahid, M. N., and Sarwar, S. (2020). Pharmacological Analysis of Obnoxious Water weed: Eichhornia crassipes (Mart.) Solms. The Japs 30 (6), 1465–1475. doi:10.36899/JAPS.2020.6.0167
Kiristos, T. G., Kebede, A., Chaithanya, K. K., and Zenebe Teka, M. (2018). Evaluation of In Vitro Antibacterial Potential of Eichhornia crassipes Leaf Extracts. Drug Discov. Today 10 (5 Suppl. l), 3824–3831.
Kumar, D., Karthik, M., and Rajakumar, R. (2018a). GC-MS Analysis of Bioactive Compounds from Ethanolic Leaves Extract of Eichhornia crassipes (Mart) Solms. And Their Pharmacological Activities. Pharma Innov. J. 7, 459–462.
Kumar, D., Karthik, M., and Rajakumar, R. (2018b). In-silico Antibacterial Activity of Active Phytocompounds from the Ethanolic Leaves Extract of Eichhornia crassipes (Mart) Solms. Against Selected Target Pathogen Pseudomonas Fluorescens. J. Pharmacogn. Phytochem. 7, 12–15.
Kumar, S., Kumar, R., Dwivedi, A., and Pandey, A. K. (2014). In VitroAntioxidant, Antibacterial, and Cytotoxic Activity andIn VivoEffect ofSyngonium podophyllumandEichhornia crassipesLeaf Extracts on Isoniazid Induced Oxidative Stress and Hepatic Markers. Biomed. Res. Int. 2014, 1–11. doi:10.1155/2014/459452
Kurniawan, F., Wongso, M., Ayucitra, A., Soetaredjo, F. E., Angkawijaya, A. E., and Ju, Y. H. (2015). Carbon Microsphere From Water Hyacinth For Supercapacitor Electrode. J. Taiwan Inst. Chem. Eng. 47, 197–201.
Lai, H., Peng, J., Liao, B., Xian, J., Zhang, Z., Guo, J., et al. (2011). Experimental Studies for Levulinic Acid Production from Water Hyacinth Plant. I.C.M.R.E.E. 1, 373–376. doi:10.1109/icmree.2011.5930833
Lalitha, P., and Jayanthi, P. (2014). Antiaging Activity of the Skin Cream Containing Ethyl Acetate Extract of Eichhornia crassipes (Mart.) Solms. Int. J. Pharmtech Res. 6, 29–34.
Lalitha, T. P., and Jayanthi, P. (2012). Preliminary Studies on Phytochemicals and Antimicrobial Activity of Solvent Extracts of Eichhornia crassipes (Mart.) Solms. Asian J. Plant Sci. Res. 2 (2), 115–122.
Lata, N., and Dubey, V. (2010). Quantification and Identification of Alkaloids of Eichhornia crassipes: the World’s Worst Aquatic Plant. J. Pharm. Res. 3, 1229–1231.
Lata, N., and Veenapani, D. (2010). Isolation of Flavonoids from Eichhornia crassipes: the World's Worst Aquatic Plant. J. Pharm. Res. 3 (9), 2116–2118.
Leconte, N., and Rossignol-Castera, A. (2014). Use of a lipophilic extract of water hyacinth for moisturising the skin. Patent No EP2777709A1. Societe de Recherche Cosmetique SARL. Luxembourg (LU): European Patent Office.
Lenora, L. M., Kumara, J. S., Murugesanb, S., and Senthilkumarb, N. (2015). Anticancer Activity of Water Hyacinth [Eichhornia crassipes (mart) Solms] on Human Cervical Cancer Cell Line. Oct. J. Env. Res. 3 (4), 327–331.
Lenora, L. M., Kumara, J. S., Murugesanb, S., and Senthilkumarb, N. (2016). GC-MS-MS Analysis of Alien Invasive Aquatic weed, Eichhornia crassipes (Mart.) Solms. Der. Chem. Sinica. 7, 48–52.
Lenora, L. M., and Senthilkumar, N. (2017). Insecticidal Potential of Aquatic Alien weed, Eichhornia crassipes (Mart) Solms on Tobacco Caterpillar, Spodoptera Litura (F.). Asian J. Plant Sci. Res. 7 (1), 1–6.
Liu, X., Zu, X., Liu, Y., Sun, L., Yi, G., Lin, W., et al. (2018). Conversion of Wastewater Hyacinth into High-Value Chemicals by Iron (III) Chloride under Mild Conditions. BioResources 13 (2), 2293–2303. doi:10.15376/biores.13.2.2293-2303
Malik, A. (2007). Environmental challenge Vis a Vis Opportunity: the Case of Water Hyacinth. Environ. Int. 33 (1), 122–138. doi:10.1016/j.envint.2006.08.004
Manivannan, A., and Narendhirakannan, R. T. (2014). Response Surface Optimization for Co-production of Cellulase and Xylanase Enzymes by Trichoderma Reesei NRRL–3652. Int. J. Chemtech. Res. 6 (7), 3883–3888.
Manyuchi, M. M., Mbohwa, C., Muzenda, E., Mutusva, T. N., and Mpeta, M. (20192019). Degradation of Water Hyacinth (Eichhornia crassipes) to Vermicompost through Application of the Vermicomposting Technology. Proc. Int. Conf. Ind. Eng. Oper. Manag. IEOM 1, 79–88. doi:10.33965/ste2019_201901l010
Martins, P. F., de Melo, M. M. R., Sarmento, P., and Silva, C. M. (2016). Supercritical Fluid Extraction of Sterols from Eichhornia crassipes Biomass Using Pure and Modified Carbon Dioxide. Enhancement of Stigmasterol Yield and Extract Concentration. J. Supercrit. Fluids 107, 441–449. doi:10.1016/j.supflu.2015.09.027
Mishra, S., and Maiti, A. (2017). The Efficiency of Eichhornia crassipes in the Removal of Organic and Inorganic Pollutants from Wastewater: a Review. Environ. Sci. Pollut. Res. Int. 24 (9), 7921–7937. doi:10.1007/s11356-016-8357-7
Mtewa, A. G., Deyno, S., Ngwira, K., Lampiao, F., Peter, E. L., Ahovegbe, L. Y., et al. (2018). Drug-like Properties of Anticancer Molecules Elucidated from. Eichhornia crassipes. J. Pharmacogn. Phytochem. 7 (5), 2075–2079.
Mustafa, H. M., and Hayder, G. (2021). Recent Studies on Applications of Aquatic weed Plants in Phytoremediation of Wastewater: A Review Article. Ain Shams Eng. J. 12 (1), 355–365. doi:10.1016/j.asej.2020.05.009
Muthunarayanan, V., Santhiya, M., Swabna, V., and Geetha, A. (2011). Phytodegradation of Textile Dyes by Water Hyacinth (Eichhornia crassipes) from Aqueous Dye Solutions. Int. J. Environ. Sci. 1 (7), 1702–1717.
Nugriani, N. O., Diah, I. W. D., and Yusri, S. (2020). Antioxidant Stability Testing on Liquid and Powder Eichhornia crassipes Extract. IOP Conf. Ser. Mater. Sci. Eng. 742 (1), 12019. doi:10.1088/1757-899x/742/1/012019
Nyananyo, B., Gijo, A., and Ogamba, E. (2007). The Physico-Chemistry and Distribution of Water Hyacinth (Eichhornia Cressipes) on the River Nun in the Niger Nelta. J. Appl. Sci. Environ. Manage. 11 (3), 133–137. doi:10.4314/jasem.v11i3.55158
Paniagua-Pérez, R., Madrigal-Bujaidar, E., Reyes-Cadena, S., Alvarez-González, I., Sánchez-Chapul, L., and Pérez-Gallaga, J. (2008). Cell Protection Induced by Beta-Sitosterol: Inhibition of Genotoxic Damage, Stimulation of Lymphocyte Production, and Determination of its Antioxidant Capacity. Arch. Toxicol. 82 (9), 615–622.
Parsons, W. T., and Cuthbertson, E. G. (2001). Noxious Weeds of Australia. Australia: CSIRO publishing.
Patel, S. (2012). Threats, Management and Envisaged Utilizations of Aquatic weed Eichhornia crassipes: An Overview. Rev. Environ. Sci. Biotechnol. 11 (3), 249–259. doi:10.1007/s11157-012-9289-4
Poomsawat, W., Tsalidis, G., Tsekos, C., and Jong, W. (2019). Experimental Studies of Furfural Production from Water Hyacinth ( Eichhornia Crassipes ). Energy Sci. Eng. 7 (5), 2155–2164. doi:10.1002/ese3.420
Pothiraj, C., Arumugam, R., and Gobinath, R. M. (2016). Production of Cellulase in Submerged Fermentation Using Water Hyacinth as Carbon Source and Reutilization of Spent Fungal Biomass for Dye Degradation. Int.J.Curr.Microbiol.App.Sci 5 (10), 99–108. doi:10.20546/ijcmas.2016.510.012
Prakasham, R. S., Rao, R. S., and Hobbs, P. J. (2009). Current Trends in Biotechnological Production of Xylitol and Future Prospects. Curr. Trends Biotechnol. Pharm. 3 (1), 8–36.
Qiu, Z., Huang, T., Zhao, C., Luo, J., and Hu, Z. (2017). Water Hyacinth‐derived Activated carbon/NiO Nanocomposite as a Facile Electrode Material for High Performance Supercapacitor. Micro Nano Lett. 12 (4), 231–235. doi:10.1049/mnl.2016.0526
Radhika, D., and Murugesan, A. G. (2012). Bioproduction, Statistical Optimization and Characterization of Microbial Plastic (Poly 3-hydroxy Butyrate) Employing Various Hydrolysates of Water Hyacinth (Eichhornia crassipes) as Sole Carbon Source. Bioresour. Technol. 121, 83–92. doi:10.1016/j.biortech.2012.06.107
Rahmatullah, M., Mollik, A. H., Rashid, H., Tanzin, R., Ghosh, K. C., Rahman, H., et al. (2010). A Comparative Analysis of Medicinal Plants Used by Folk Medicinal Healers in Villages Adjoining the Ghaghot, Bangali and Padma Rivers of Bangladesh. Am-eurasian. J. Sustain. Agric. 4, 70–85.
Raj, K., and Krishnan, C. (2020). Improved Co-production of Ethanol and Xylitol from Low-Temperature Aqueous Ammonia Pretreated Sugarcane Bagasse Using Two-Stage High Solids Enzymatic Hydrolysis and Candida tropicalis. Renew. Energ. 153, 392–403. doi:10.1016/j.renene.2020.02.042
Rop, K., Mbui, D., Karuku, G. N., Michira, I., and Njomo, N. (2020). Characterization of Water Hyacinth Cellulose-G-Poly(ammonium Acrylate-Co-Acrylic Acid)/nano-Hydroxyapatite Polymer Hydrogel Composite for Potential Agricultural Application. Results Chem. 2, 100020. doi:10.1016/j.rechem.2019.100020
Rorong, J. A., Sudiarso, S., Prasetya, B., Polii-Mandang, J., and Suryanto, E. (2012). Phytochemical Analysis of Eceng Gondok (Eichhornia crassipes Solms) of Agricultural Waste as Biosensitizer for Ferri Photoreduction. Agrivita, J. Agric. Sci. 34 (2), 152–160. doi:10.17503/agrivita-2012-34-2-p152-160
Rufchaei, R., Mirvaghefi, A., Hoseinifar, S. H., Valipour, A., and Nedaei, S. (2020). Effects of Dietary Administration of Water Hyacinth (Eichhornia crassipes) Leaves Extracts on Innate Immune Parameters, Antioxidant Defence and Disease Resistance in Rainbow trout (Oncorhynchus mykiss). Aquaculture 515, 734533. doi:10.1016/j.aquaculture.2019.734533
Rufchaie, R., Mirvaghefi, A., Hoseinifar, S. H., and Valipour, A. (2018). Anti-microbial Activity of Echhornia Crasippes Aquatic and Hydromethanolic Leaves Extract. J. Fish. 71 (1), 31–41.
Saning, A., Herou, S., Dechtrirat, D., Ieosakulrat, C., Pakawatpanurut, P., Kaowphong, S., et al. (2019). Green and Sustainable Zero-Waste Conversion of Water Hyacinth (Eichhornia crassipes) into superior Magnetic Carbon Composite Adsorbents and Supercapacitor Electrodes. RSC Adv. 9, 24248–24258. doi:10.1039/c9ra03873f
Saratale, R. G., Cho, S. K., Ghodake, G. S., Shin, H. S., Saratale, G. D., and Park, Y. (2020). Utilization of Noxious Weed Water Hyacinth Biomass As A Potential Feedstock For Biopolymers Production: A Novel Approach. Polymers 12 (8), 1704
Setyaningsih, L., Satria, E., Khoironi, H., Dwisari, M., Setyowati, G., and Rachmawati, N. (2019). Cellulose Extracted From Water Hyacinth And The Application In Hydrogel. In IOP Conference Series: Materials Science and Engineering 673 (1), 012017IOP Publishing
Shanab, S. M., Shalaby, E. A., Lightfoot, D. A., and El-Shemy, H. A. (2010). Allelopathic Effects of Water Hyacinth [Eichhornia crassipes]. PLoS ONE 5 (10), e13200. doi:10.1371/journal.pone.0013200
Shanab, S. M. M., Ameer, M. A., Fekry, A. M., Ghoneim, A. A., and Shalaby, E. A. (2011). Corrosion Resistance of Magnesium alloy (AZ31E) as Orthopaedic Biomaterials in Sodium Chloride Containing Antioxidantly Active Compounds from Eichhornia crassipes. Int. J. Electrochem. Sci. 6, 3017–3035.
Shanab, S. M. M., and Shalaby, E. A. (2012). Biological Activities and Anticorrosion Efficiency of Water Hyacinth (Eichhornia crassipes). J. Med. Plant Res. 6 (23), 3950–3962. doi:10.5897/JMPR12.1910.5897/jmpr12.191
Shankar, K., Kulkarni, N. S., Sajjanshetty, R., Jayalakshmi, S. K., and Sreeramulu, K. (2020). Co-production of Xylitol and Ethanol by the Fermentation of the Lignocellulosic Hydrolysates of Banana and Water Hyacinth Leaves by Individual Yeast Strains. Ind. Crops Prod. 155, 112809. doi:10.1016/j.indcrop.2020.112809
Shanyuan, Y., Ziwen, Y. U., Wenhao, S. U. N., and Houming, W. (1992). Isolation and Identification of Antialgal Compounds from Root System of Water Hyacinth (Eichhornia crassipes). J. .Plants. Physiol. Mol. Biol. 18 (4), 399–402.
Sharma, A. K., Sharma, V., Sharma, V., Sharma, J. K., and Singh, R. (2020). Multifaceted Potential of Eichhornia crassipes (Water Hyacinth) Ladened with Numerous Value Aided and Therapeutic Properties. Plant Arch. 20 (2), 2059–2065.
Shehnaz, B. T., and Vijayalakshmi, M. (2016). Antibacterial Activity of Methanolic Extract of the Flowers of Eichhornia crassipes (Mart.) Solms. Against Two Different Strains of Bacteria. Int. J. Pharm. Bio. Sci. 7, 1088–1091.
Silva, R. P., de Melo, M. M. R., Silvestre, A. J. D., and Silva, C. M. (2015). Polar and Lipophilic Extracts Characterization of Roots, Stalks, Leaves and Flowers of Water Hyacinth (Eichhornia crassipes), and Insights for its Future Valorization. Ind. Crops Prod. 76, 1033–1038. doi:10.1016/j.indcrop.2015.07.055
Sindhu, R., Binod, P., Pandey, A., Madhavan, A., Alphonsa, J. A., Vivek, N., et al. (2017). Water Hyacinth a Potential Source for Value Addition: an Overview. Bioresour. Technol. 230, 152–162. doi:10.1016/j.biortech.2017.01.035
Singh, K. S., Sawant, S. G., Devi, P., and Kaminsky, W. (2015). Stigmasterol from Eichhornia crassipes (Water Hyacinth): Isolation, Characterization and X-ray Structure. Asian J. Chem. 27 (8), 3028–3030. doi:10.14233/ajchem.2015.18832
Soenjaya, S. A., Handoyo, N., Edi Soetaredjo, F., Angkawijaya, A. E., Ju, Y.-H., and Ismadji, S. (2015). Preparation of Carbon Fiber from Water Hyacinth Liquid Tar. Int. J. Ind. Chem. 6, 1–7. doi:10.1007/s40090-014-0026-4
Su, W., Sun, Q., Xia, M., Wen, Z., and Yao, Z. (2018). The Resource Utilization of Water Hyacinth (Eichhornia crassipes [Mart.] Solms) and its Challenges. Resources 7 (3), 46. doi:10.3390/resources7030046
Sun, W.-H., Yu, Z.-W., Tai, G.-F., and Yu, S.-W. (1990). Sterilized Culture of Water Hyacinth and its Application in the Study of Allelopathic Effect [s] on Algae. Acta Phytophysiol. Sin. 16 (3), 301–305.
Sunitha, P., Apparao, S., Sandhya, R. M., Sirisha, B., and Lavanya, K. (2018). Evaluation of Antibacterial, Anti-inflammatory and Antioxidant Activities of Methanolic Extract of Whole Plant of. Eichhornia crassipes. Int. J. Pharm. Sci. Rev. Res. 48 (1), 37–42.
Surendraraj, A., Farvin, K. H. S., and Anandan, R. (2013). Antioxidant Potential of Water Hyacinth (Eichornia Crassipes): In Vitro Antioxidant Activity and Phenolic Composition. J. Aquat. Food Product. Technology 22 (1), 11–26. doi:10.1080/10498850.2011.621582
Tan, D. X., Manchester, L. C., Di Mascio, P., Martinez, G. R., Prado, F. M., and Reiter, R. J. (2007). Novel Rhythms of N 1 ‐acetyl‐N 2 ‐formyl‐5‐methoxykynuramine and its Precursor Melatonin in Water Hyacinth: Importance for Phytoremediation. FASEB j. 21 (8), 1724–1729. doi:10.1096/fj.06-7745com
Taqi, Z. J., Hamad ohammed, A., and Jabir, M. S. (2019). Biomedical Applications of Eichhornia crassipes. Res. J. Biotech. 14 (Special Issue I), 156–159.
Téllez, T. R., López, E., Granado, G., Pérez, E., López, R., and Guzmán, J. (2008). The Water Hyacinth, Eichhornia crassipes: an Invasive Plant in the Guadiana River Basin (Spain). Ai 3 (1), 42–53. doi:10.3391/ai.2008.3.1.8
Thamaraiselvi, P., and Jayanthi, P. (2012). Preliminary Studies on Phytochemicals and Antimicrobial Activity of Solvent Extracts of Eichhornia crassipes (Mart.) Solms. Asian J. Plant Sci. Res. 2 (2), 115–122.
Thiripura Sundari, M., and Ramesh, A. (2012). Isolation and Characterization of Cellulose Nanofibers from the Aquatic weed Water Hyacinth-Eichhornia crassipes. Carbohydr. Polym. 87 (2), 1701–1705. doi:10.1016/j.carbpol.2011.09.076
Thombre, R., Chitnis, A., Kadam, V., Bogawat, Y., Colaco, R., and Kale, A. (2014). A Facile Method for Synthesis of Biostabilized Silver Nanoparticles Using Eichhornia crassipes (Mart.) Solms (Water Hyacinth). Indian J. Biotechnol. 13, 337–341.
Toki, K., Saito, N., Iimura, K., Suzuki, T., and Honda, T. (1994). (Delphinidin 3-gentiobiosyl) (Apigenin 7-glucosyl) Malonate from the Flowers of Eichhornia crassipes. Eichhornia crassipes. Phytochemistry 36 (5), 1181–1183. doi:10.1016/s0031-9422(00)89634-x
Tulika, T., and Mala, A. (2015). Pharmaceutical Potential of Aquatic Plant Pistia stratiotes (L.) and. Eichhornia crassipes. J. Plant Sci. 3 (1–1), 10–18. doi:10.11648/j.jps.s.2015030101.12
Turnipseed, R. K., Moran, P. J., and Allan, S. A. (2018). Behavioral Responses of Gravid Culex quinquefasciatus, Aedes aegypti, and Anopheles quadrimaculatus Mosquitoes to Aquatic Macrophyte Volatiles. J. Vector Ecol. 43 (2), 252–260. doi:10.1111/jvec.12309
Tyagi, T., and Agarwal, M. (2017b). Antioxidant Properties and Phenolic Compounds in Methanolic Extracts of Eichhornia crassipes. Res. J. Phytochemistry 11 (2), 85–89. doi:10.3923/rjphyto.2017.85.89
Tyagi, T., and Agarwal, M. (2017a). GC-MS Analysis of Invasive Aquatic Weed, Pistia Stratiotes L. And Eichhornia crassipes (Mart.) Solms. Int. J. Curr. Pharm. Sci. 9 (3), 111–117. doi:10.22159/ijcpr.2017.v9i3.19970
Udeh, C. B., Ameh, J. B., Ado, S. A., and Okoduwa, S. I. R. (2017). Optimization of Xylanase Production from Fermentation of Water Hyacinth (Eichhornia crassipes) Using Trichoderma Species. J. Biotechnol. Res. 3 (3), 15–24.
Virabalin, R., Kositsup, B., and Punnapayak, H. (1993). Leaf Protein Concentrate from Water Hyacinth. J. Aquat. Plant Manage. 31, 207–209.
Wali, S., ur Rehman, K., Ullah, B., Yaseen, T., and Ahmad, G. (2019). Efficiency of Common Water Hyacinth (Eichhornia crassipes) in Controlling Growth of Fungal and Bacterial Clinical Strains. Pab 8 (4), 2178–2186. doi:10.19045/bspab.2019.80163
Wang, S. (2015). Eichhornia crassipes Hand creamPatent No CN104415177A. China: Qingdao Medical Prevention Disinfection Professional Technology Center.
Wang, Z., Liu, J., Ning, Y., Liao, X., and Jia, Y. (2017). Eichhornia crassipes: Agro-Waster for a Novel Thermostable Laccase Production by Pycnoporus Sanguineus SYBC-L1. J. Biosci. Bioeng. 123 (2), 163–169. doi:10.1016/j.jbiosc.2016.09.005
Wasowicz, E., and Rudzinska, M. (2011). Cholesterol and Phytosterols. Chem. Biol. Funct. Asp. Food Lipids 1, 113–134. doi:10.1201/b10272-8
Wu, W., Guo, X., and Huang, M. (2014). Evaluation of Acute Toxicity Potential of Water Hyacinth Leaves. Toxicol. Ind. Health 30 (5), 426–431. doi:10.1177/0748233712458138
Wu, X., Zhang, Z., Chen, D., Zhang, J., Yang, W., and Jin, Y. (2012). Allelopathic Effects of Eichhornia crassipes on the Growth of. Microcystis Aeruginosa. J. Agric. Sci. Technol. A. 2 (12A), 1400.
Yan, S. H., Song, W., and Guo, J. Y. (2017). Advances in Management and Utilization of Invasive Water Hyacinth (Eichhornia crassipes) in Aquatic Ecosystems - a Review. Crit. Rev. Biotechnol. 37 (2), 218–228. doi:10.3109/07388551.2015.1132406
Yu, B., Yang, L., and Zeng, H. F. Z. (2020). Extraction Process, Optimization Method and Application of Triterpenoids in Eyeichhornia CrassipesPatent No CN112076237A. China: Hainan Medical College.
Zhang, Y., Shen, Y., Zhang, H., Wang, L., Zhang, H., Qian, H., et al. (2018). Isolation, Purification and Identification of Two Antioxidant Peptides from Water Hyacinth Leaf Protein Hydrolysates (WHLPH). Eur. Food Res. Technol. 244 (1), 83–96. doi:10.1007/s00217-017-2941-z
Zhang, Y. Y., Zhang, D. Y., and Barrett, S. C. (2010). Genetic Uniformity Characterizes the Invasive Spread of Water Hyacinth (Eichhornia crassipes), a Clonal Aquatic Plant. Mol. Ecol. 19 (9), 1774–1786. doi:10.1111/j.1365-294X.2010.04609.x
Zhao, S., Liang, X., Hua, D., Ma, T., and Zhang, H. (2011). High-yield Cellulase Production in Solid-State Fermentation by Trichoderma Reesei SEMCC-3.217 Using Water Hyacinth (Eichhornia crassipes). Afr. J. Biotechnol. 10 (50), 10178–10187.
Keywords: Eichhornia crassipes (mart.) Solms, phytochemistry, value–added products, pharmacology, biological activities
Citation: Ben Bakrim W, Ezzariai A, Karouach F, Sobeh M, Kibret M, Hafidi M, Kouisni L and Yasri A (2022) Eichhornia crassipes (Mart.) Solms: A Comprehensive Review of Its Chemical Composition, Traditional Use, and Value-Added Products. Front. Pharmacol. 13:842511. doi: 10.3389/fphar.2022.842511
Received: 23 December 2021; Accepted: 23 February 2022;
Published: 18 March 2022.
Edited by:
Ilkay Erdogan Orhan, Gazi University, TurkeyReviewed by:
Hazel Monica M. Peralta, Central Luzon State University, PhilippinesFardous Mohammad Safiul Azam, Neijiang Normal University, China
Copyright © 2022 Ben Bakrim, Ezzariai, Karouach, Sobeh, Kibret, Hafidi, Kouisni and Yasri. This is an open-access article distributed under the terms of the Creative Commons Attribution License (CC BY). The use, distribution or reproduction in other forums is permitted, provided the original author(s) and the copyright owner(s) are credited and that the original publication in this journal is cited, in accordance with accepted academic practice. No use, distribution or reproduction is permitted which does not comply with these terms.
*Correspondence: Mohamed Hafidi, hafidi@uca.ac.ma